Gut flora
Editor-In-Chief: C. Michael Gibson, M.S., M.D. [1]
Overview
WikiDoc Resources for Gut flora |
Articles |
---|
Most recent articles on Gut flora |
Media |
Evidence Based Medicine |
Clinical Trials |
Ongoing Trials on Gut flora at Clinical Trials.gov Clinical Trials on Gut flora at Google
|
Guidelines / Policies / Govt |
US National Guidelines Clearinghouse on Gut flora
|
Books |
News |
Commentary |
Definitions |
Patient Resources / Community |
Patient resources on Gut flora Discussion groups on Gut flora Directions to Hospitals Treating Gut flora Risk calculators and risk factors for Gut flora
|
Healthcare Provider Resources |
Causes & Risk Factors for Gut flora |
Continuing Medical Education (CME) |
International |
|
Business |
Experimental / Informatics |
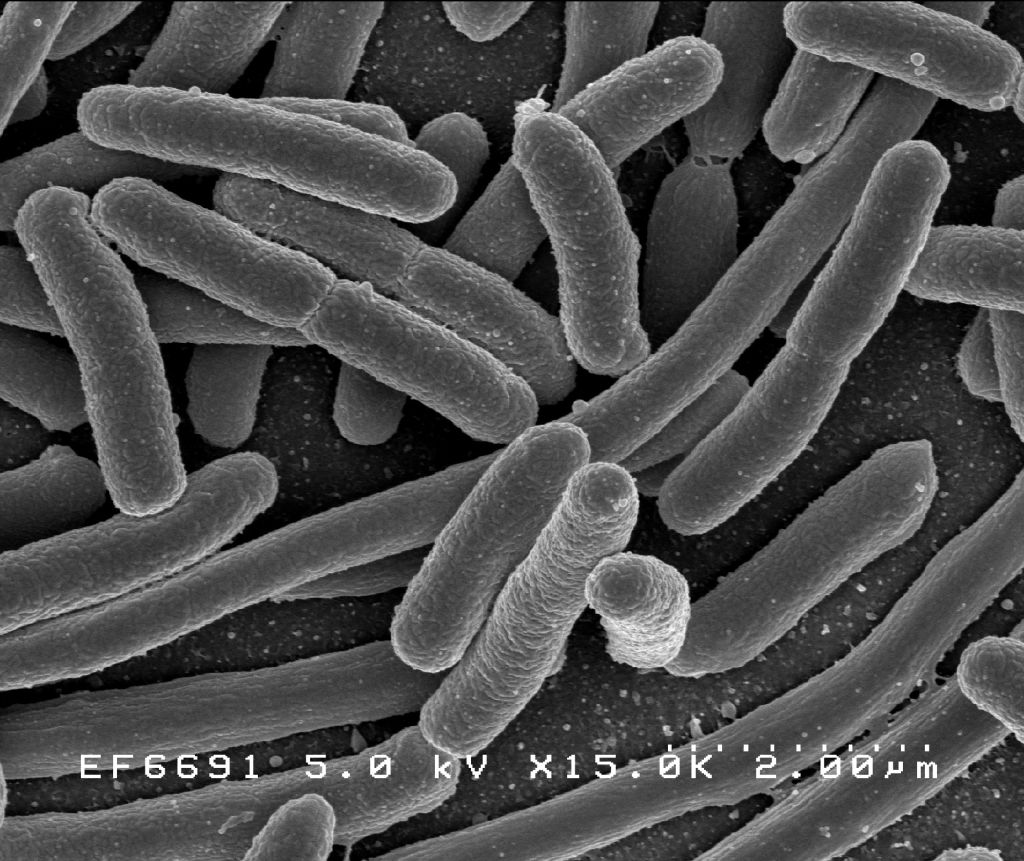
The gut flora are the microorganisms that normally live in the digestive tract and can perform a number of useful functions for their hosts.
The average human body, consisting of about 1013 cells, has about ten times that number of microorganisms in the gut.[1][2][3][4][5] Bacteria make up most of the flora in the colon[5] and 60% of the mass of feces.[2] Somewhere between 300[2] and 1000 different species live in the gut,[3] with most estimates at about 500.[6][4] However, it is probable that 99% of the bacteria come from about 30 or 40 species.[7] Fungi and protozoa also make up a part of the gut flora, but little is known about their activities.
Research suggests that the relationship between gut flora and humans is not merely commensal (a non-harmful coexistence), but rather is a mutualistic, symbiotic relationship.[3] Though people can survive with no gut flora,[4] the microorganisms perform a host of useful functions, such as fermenting unused energy substrates, training the immune system, preventing growth of harmful species,[2] regulating the development of the gut, producing vitamins for the host (such as biotin and vitamin K), and producing hormones to direct the host to store fats. However, in certain conditions, some species are thought to be capable of causing disease by causing infection or increasing cancer risk for the host.[2][5]
Localization
The colon has the greatest numbers of bacteria and the most different species, and the activity of these bacteria make the colon the most metabolically active organ in the body.[6] Most of the bacteria in the small intestine are Gram-positive, while those in the colon are mostly Gram-negative.[8] The first part of the colon is mostly responsible for fermenting carbohydrates,[7][6][2] while the latter part mostly breaks down proteins and amino acids.[6][2] Bacterial growth is rapid in the cecum and ascending colon, which has a low pH, and slow in the descending colon, which has an almost neutral pH.[2] The body maintains the proper balance and locations of species by altering pH, the activity of the immune system, and peristalsis.[5]
Over 99% of the bacteria in the gut are anaerobes,[7][2][5][3][9] but in the cecum aerobic bacteria reach high densities.[2]
Types
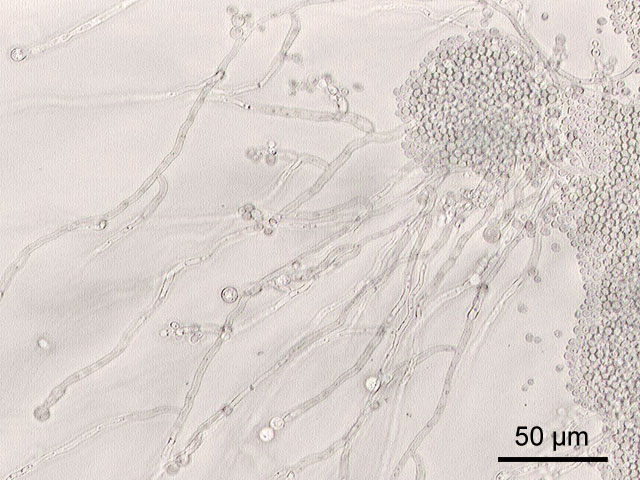
Not all the species in the gut have been identified[2][3] because some cannot be cultured,[7][3][10] so DNA isolation and identification is difficult.[11] Populations of species vary widely among different individuals but stay fairly constant within an individual over time.[2]
Most bacteria come from the genera Bacteroides, Clostridium, Fusobacterium,[7][2][9] Eubacterium, Ruminococcus, Peptococcus, Peptostreptococcus, and Bifidobacterium.[2][7] Other genera such as Escherichia and Lactobacillus are present to a lesser extent.[2] Species from the genus Bacteroides alone constitute about 30% of all bacteria in the gut, suggesting that that genus is especially important in the functioning of the host.[3]
The currently known genera of fungi of the gut flora include Candida, Saccharomyces, Aspergillus, and Penicillium.
Acquisition of gut flora in human infants
The gastrointestinal tract of a normal fetus is sterile. During birth and rapidly thereafter, bacteria from the mother and the surrounding environment colonize the infant gut. Immediately after vaginal delivery, babies have bacterial strains in the upper gastrointestinal tract derived from the mothers’ feces.[12] Infants born by caesarean section may also be exposed to their mothers’ microflora, but the main exposure is from the surroundings.[13] After birth, environmental, oral and cutaneous bacteria are readily transferred from the mother to the infant through suckling, kissing, and caressing. All infants are initially colonized by large numbers of E. coli and streptococci. Within a few days, bacterial numbers reach 108 – 1010 /g feces.[13][14] During the first week of life, these bacteria create a reducing environment favorable for the subsequent bacterial succession of strict anaerobic species mainly belonging to the genera Bifidobacterium, Bacteroides, Clostridium, and Ruminococcus.[15] Breast-fed babies become dominated by bifidobacteria, possibly due to the contents of bifidobacterial growth factors in breast milk.[16] In contrast, the microflora of formula-fed infants is more diverse with high numbers of Enterobacteriaceae, enterococci, bifidobacteria, Bacteroides, and clostridia.[17][18] After the introduction of solid food and weaning, the microflora of breast-fed infants becomes similar to that of formula-fed infants. By the second year of life the fecal microflora resembles that of adults.
Functions
Bacteria in the gut fulfills a host of useful functions for humans, including digestion of unutilized energy substrates;[19] stimulating cell growth; repressing the growth of harmful microorganisms; training the immune system to respond only to pathogens; and defending against some diseases.[2][3][20]
Carbohydrate fermentation and absorption
Without gut flora, the human body would be unable to utilize some of the undigested carbohydrates it consumes, because some types of gut flora have enzymes that human cells lack for breaking down certain polysaccharides.[3] Rodents raised in a sterile environment and lacking in gut flora need to eat 30% more calories just to remain the same weight as their normal counterparts.[3] Carbohydrates that humans cannot digest without bacterial help include certain starches; fiber; oligosaccharides and sugars that the body failed to digest and absorb[6][2][7] like lactose and sugar alcohols, mucus produced by the gut, and proteins.[6]
Bacteria turn carbohydrates they ferment into short chain fatty acids, or SCFAs.[6][5][7] These materials can be used by host cells, providing a major source of useful energy and nutrients for humans.[6] They increase the gut's absorption of water, reduce counts of damaging bacteria, increase growth of human gut cells,[5] and are also used for the growth of indigenous bacteria.[2] The SCFAs are produced by a form of fermentation called saccharolytic fermentation[6] and include acetic acid, propionic acid, and butyric acid.[6][5][7] Gases and organic acids like lactic acid are also produced by saccahrolytic fermentation.[7] Acetic acid is used by muscle, propionic acid helps the liver produce ATP, and butyric acid provides energy to gut cells and may prevent cancer.[6]
Another, less favorable type of fermentation, proteolytic fermentation, breaks down proteins like enzymes, dead host and bacterial cells, and collagen and elastin found in food, and can produce toxins and carcinogens in addition to SCFAs. Thus a diet lower in protein lowers exposure to toxins.[2][5]
Evidence also suggests that bacteria enhance the absorption and storage of lipids.[3] Bacteria also produce and help the body absorb needed vitamins like vitamin K. In addition, the SCFAs they produce help the body absorb nutrients such as calcium, magnesium, and iron.[2]
Trophic effects
Another benefit of SCFAs is that they increase growth of intestinal epithelial cells and control their proliferation and differentiation.[2] They may also cause lymphoid tissue near the gut to grow. Bacterial cells also alter intestinal growth by changing the expression of cell surface proteins such as sodium/glucose transporters.[3] In addition, changes they make to cells may prevent injury to the gut mucosa from occurring.[20]
Repression of pathogenic microbial growth
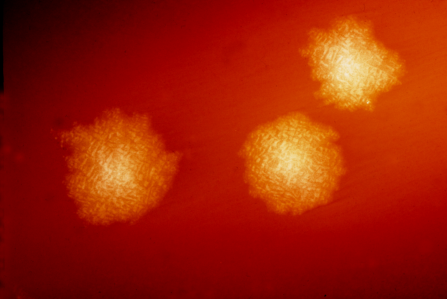
Another important role of helpful gut flora is that they prevent species that would harm the host from colonizing the gut, an activity termed the "barrier effect". Yeasts and harmful bacterial species such as Clostridium difficile (the overgrowth of which can cause pseudomembranous colitis) are unable to grow too much due to competition from helpful gut flora species, thus animals without gut flora are infected very easily. The barrier effect protects humans from both invading species and species normally present in the gut at low numbers, whose growth is usually inhibited by the gut flora.[2]
Helpful bacteria prevent the growth of pathogenic species by competing for nutrition and attachment sites to the epithelium of the colon. Symbiotic bacteria are more at home in this ecological niche and are thus more successful in the competition. The indigenous bacteria send chemical signals to the host about the amount of nutrients they need, and the host provides only that much, so harmful bacteria are starved out. Indigenous gut flora also produce bacteriocins, substances which kill harmful microbes and the levels of which can be regulated by enzymes produced by the host.[2]
The process of fermentation, since it produces fatty acids, also serves to lower the pH in the colon, preventing the proliferation of harmful species of bacteria and facilitating that of helpful species. The pH may also enhance the excretion of carcinogens.[6]
Immunity
Gut flora have a continuous and dynamic effect on the host's gut and systemic immune systems. The bacteria are key in promoting the early development of the gut's mucosal immune system both in terms of its physical components and function and continue to play a role later in life in its operation. The bacteria stimulate the lymphoid tissue associated with the gut mucosa to produce antibodies to pathogens. The immune system recognizes and fights harmful bacteria, but leaves the helpful species alone, a tolerance developed in infancy.[2][10][4][5]
As soon as an infant is born, bacteria begin colonizing its digestive tract. The first bacteria to settle in are able to affect the immune response, making it more favorable to their own survival and less so to competing species; thus the first bacteria to colonize the gut are important in determining the person's lifelong gut flora makeup. However, there is a shift at the time of weaning from predominantly facultative aerobic species such as Streptococci and Escherichia coli to mostly obligate anaerobic species.[2][3]
Recent findings have shown that gut bacteria play a role in the expression of Toll-like receptors (TLRs) in the intestines, molecules that help the host repair damage due to injury. TLRs cause parts of the immune system to repair injury caused for example by radiation.[3][20]
Bacteria can influence the phenomenon known as oral tolerance, in which the immune system is less sensitive to an antigen (including those produced by gut bacteria) once it has been ingested. This tolerance, mediated in part by the gastrointestinal immune system and in part by the liver, can reduce an overreactive immune response like those found in allergies and auto-immune disease.[21]
Some species of gut flora, such as some of those in the Bacteroides genus, are able to change their surface receptors to mimic those of host cells in order to evade immune response. Bacteria with neutral and harmful effects on the host can also use these types of strategies. The host immune system has also adapted to this activity, preventing overgrowth of harmful species.[2][4]
Preventing allergy
Bacteria are also implicated in preventing allergies,[1] an overreaction of the immune system to non-harmful antigens. Studies on the gut flora of infants and young children have shown that those who have or later develop allergies have different compositions of gut flora from those without allergies, with higher chances of having the harmful species C difficile and S aureus and lower prevalence of Bacteroides and Bifidobacteria.[1] One explanation is that since helpful gut flora stimulate the immune system and "train" it to respond properly to antigens, a lack of these bacteria in early life leads to an inadequately trained immune system which overreacts to antigens.[1] On the other hand, the differences in flora could be a result, not a cause, of the allergies.[1]
Preventing inflammatory bowel disease
Another indicator that bacteria help train the immune system is the epidemiology of Inflammatory Bowel Disease, or IBD, such as Crohn's Disease (CD). Some authors suggest that SCFAs prevent IBD. In addition, some forms of bacteria can prevent inflammation.[22] The incidence and prevalence of IBD is high in industrialized countries with a high standard of living and low in less economically developed countries, having increased in developed countries throughout the twentieth century. The disease is also linked to good hygiene in youth; lack of breastfeeding; and consumption of large amounts of sucrose and animal fat.[22] Its incidence is inversely linked with poor sanitation during the first years of life and consumption of fruits, vegetables, and unprocessed foods.[22] Also, the use of antibiotics, which kill native gut flora and harmful infectious pathogens alike, especially during childhood, is associated with inflammatory bowel disease.[19] On the other hand, using probiotics, bacteria consumed as part of the diet that impart health benefits (aside from just nutrition), helps treat IBD.
Alterations in balance
Effects of antibiotic use
Altering the numbers of gut bacteria, for example by taking broad-spectrum antibiotics, may affect the host's health and ability to digest food.[23] People may take the drugs to cure bacterial illnesses or may unintentionally consume significant amounts of antibiotics by eating the meat of animals to which they were fed.[23] Antibiotics can cause antibiotic-associated diarrhea (AAD) by irritating the bowel directly, changing the levels of gut flora, or allowing pathogenic bacteria to grow.[7] Another harmful effect of antibiotics is the increase in numbers of antibiotic-resistant bacteria found after their use, which, when they invade the host, cause illnesses that are difficult to treat with antibiotics.[23]
Changing the numbers and species of gut flora can reduce the body's ability to ferment carbohydrates and metabolize bile acids and may cause diarrhea. Carbohydrates that are not broken down may absorb too much water and cause runny stools, or lack of SCFAs produced by gut flora could cause the diarrhea.[7]
A reduction in levels of native bacterial species also disrupts their ability to inhibit the growth of harmful species such as C. difficile and Salmonella kedougou, and these species can get out of hand, though their overgrowth may be incidental and not be the true cause of diarrhea.[7][23][2]
Gut flora composition also changes in severe illnesses, due not only to antibiotic use but also to such factors as ischemia of the gut, failure to eat, and immune compromise. Negative effects from this have led to interest in selective digestive tract decontamination (SDD), a treatment to kill only pathogenic bacteria and allow the reestablishment of healthy ones.[24]
Probiotics & Prebiotics
Since the lack of gut flora can have such harmful health effects, the use of probiotics has anti-inflammatory effects in the gut and may be useful for improving health. Prebiotics are dietary components that can help foster the growth of microorganisms in the gut, which may lead to better health.[22]
Role in disease
Bacteria in the digestive tract have pathogenic properties in addition to their health-inducing ones: they can produce toxins and carcinogens[5] and have been implicated in such conditions as multisystem organ failure, sepsis, colon cancer, and IBD.[2] A major factor in health is the balance of bacterial numbers; if the numbers grow too high or low, it will result in harm to the host. The host has enzymes to regulate this balance.[5]
Cancer
Some genera of bacteria, such as Bacteroides and Clostridium, have been associated with an increase in tumor growth rate, while other genera like Lactobacillus and Bifidobacteria are known to prevent tumor formation.[2]
Translocation
Helpful bacteria can be very harmful to the host if they get outside of the intestinal tract.[3][5][9] Translocation, which occurs when bacteria leave the gut through its mucosal lining, the border between the lumen of the gut and the inside of the body,[4][25] can occur in a number of different diseases.[9][22] It can be caused by too much growth of bacteria in the small intestine, reduced immunity of the human, or increased gut lining permeability.[22] The gut can become more permeable in diseases like cirrhosis, which is damaging due in part to the activity of gut flora.[26]
If the gut is perforated, bacteria can invade the body, causing a potentially fatal infection. Aerobic bacteria can make infection by anaerobes worse by using up all available oxygen and creating an environment favorable to anaerobes.[9]
Inflammatory bowel disease
Some suspect that IBD is due to a reduction in immune tolerance and subsequent overreaction of the host's immune system to harmful or non-harmful bacteria. IBD may be caused by all of the gut flora together or some specific types.[19][27]
It has been noted that though Ulcerative Colitis and Crohn's disease (two types of IBD) probably have genetic components, they are not inherited in a Mendelian fashion and are thus probably due to a complex set of factors rather than solely to a gene.[27] Though neither bacterial colonization nor genetics is sufficient to cause the disease, bacteria probably play a role in these disorders.[27]
Some suspect that inflammation in IBD is due to increased permeability of the inner lining of the colon, which may allow bacteria to invade the tissues and cause an immune reaction that leads prolonged inflammation.[4][25] Abnormal tight junctions, which are supposed to prevent permeability, have been found in cells of patients with IBD.[25] Because of the potentially harmful role of these bacteria, antibiotics are frequently prescribed to treat Crohn’s disease.[20] However, inflammation could occur first and cause the increased intestinal permeability found in diseases such as Crohn's, so the causative role of bacteria is not clear.[25]
Colitis
It has been suggested that commensal bacteria are responsible for the development of colitis, since mice raised in a sterile environment do not get the disease.[28] However, while some bacterial strains such as C. difficile[22] and even normal gut bacteria cause colitis,[28] others prevent the disease in mice.[22]
Obesity
It is known from experiments on mice that obese mice lacking leptin, a lipid metabolism regulator (ob/ob mice), have a distinct gut flora compared to (normal) lean mice, reflected in a change in the ratio between bacteria from the divisions bacteroidetes and firmicutes, which is shifted towards less bacteroidetes and more firmicutes in obese mice.
The microbes occupying the human gut are also in direct relation to obesity. A shift in the ratio between bacterial-divisions firmicutes and bacteroidetes can be observed in lean and obese individuals – in latter a shift towards firmicutes can be observed. The ratio between firmicutes and bacteroidetes dynamically reflects the overall weight-condition of an individual, shifting towards bacteroides if an obese individual loses weight.
The mutual influence of gut flora composition and weight-condition is connected to differences in the energy-resorption potential of different ratios of firmicutes and bacteroidetes, especially in the digestion of fatty acids and dietary polysaccharides, as shown by experiments wherein the (caecum) gut flora of obese mice was transplanted into germ free recipient mice, leading to an increase in weight despite an decrease in food consumption.[29][30][31][32]
Sources and notes
- ↑ 1.0 1.1 1.2 1.3 1.4 Björkstén B, Sepp E, Julge K, Voor T, and Mikelsaar M. 2001. Allergy development and the intestinal microflora during the first year of life.] Journal of Allergy and Clinical Immunology, Volume 108, Issue 4, Pages 516-520. PMID 11590374. Accessed September 15, 2007
- ↑ 2.00 2.01 2.02 2.03 2.04 2.05 2.06 2.07 2.08 2.09 2.10 2.11 2.12 2.13 2.14 2.15 2.16 2.17 2.18 2.19 2.20 2.21 2.22 2.23 2.24 2.25 2.26 2.27 2.28 Guarner F and Malagelada JR. 2003. Gut flora in health and disease. The Lancet, Volume 361, Issue 9356, 8 February 2003, Pages 512-519. PMID 12583961. Accessed September 15, 2007
- ↑ 3.00 3.01 3.02 3.03 3.04 3.05 3.06 3.07 3.08 3.09 3.10 3.11 3.12 3.13 3.14 Sears CL. 2005. A dynamic partnership: Celebrating our gut flora. Anaerobe, Volume 11, Issue 5, Pages 247-251. PMID 16701579. Accessed September 15, 2007
- ↑ 4.0 4.1 4.2 4.3 4.4 4.5 4.6 Steinhoff U. 2005. Who controls the crowd? New findings and old questions about the intestinal microflora. Immunology Letters, Volume 99, Issue 1, 15 June , Pages 12-16. PMID 15894105. Accessed September 15, 2007
- ↑ 5.00 5.01 5.02 5.03 5.04 5.05 5.06 5.07 5.08 5.09 5.10 5.11 5.12 University of Glasgow. 2005. The normal gut flora. Available through web archive. Accessed December 26, 2006
- ↑ 6.00 6.01 6.02 6.03 6.04 6.05 6.06 6.07 6.08 6.09 6.10 6.11 Gibson RG. 2004. Fibre and effects on probiotics (the prebiotic concept). Clinical Nutrition Supplements, Volume 1, Issue 2, Pages 25-31.
- ↑ 7.00 7.01 7.02 7.03 7.04 7.05 7.06 7.07 7.08 7.09 7.10 7.11 7.12 Beaugerie L and Petit JC. 2004. Microbial-gut interactions in health and disease. Antibiotic-associated diarrhoea. Best Practice & Research Clinical Gastroenterology, Volume 18, Issue 2, Pages 337-352. PMID 15123074. Accessed September 15, 2007
- ↑ Riordan SM, McIver CJ, Wakefield D, Duncombe VM, Thomas MC, and Bolin TD. 2001. Small intestinal mucosal immunity and morphometry in luminal overgrowth of indigenous gut flora. The American Journal of Gastroenterology, Volume 96, Issue 2, Pages 494-500. PMID 11232696. Accessed September 15, 2007
- ↑ 9.0 9.1 9.2 9.3 9.4 Vedantam G and Hecht DW. 2003. Antibiotics and anaerobes of gut origin. Current Opinion in Microbiology, Volume 6, Issue 5, Pages 457-461. PMID 14572537. Accessed September 15, 2007
- ↑ 10.0 10.1 Shanahan F. 2002. The host–microbe interface within the gut. Best Practice & Research Clinical Gastroenterology, Volume 16, Issue 6, Pages 915-931. PMID 12473298. Accessed September 15, 2007
- ↑ Nordgård L, Traavik T, and Nielsen KM. 2005. Nucleic acid isolation from ecological samples—vertebrate gut flora. Methods in Enzymology, Volume 395, Pages 38-48. PMID 15865959. Accessed September 7, 2007
- ↑ Bettelheim KA, Breadon A, Faiers MC, O'Farrell SM, Shooter RA. 1974. The origin of O serotypes of Escherichia coli in babies after normal delivery. Journal of Hygeine, Volume 72, Issue 1, Pages 67-70. PMID 4593741. Accessed September 3, 2007
- ↑ 13.0 13.1 Schwiertz A, Gruhl B, Lobnitz M, Michel P, Radke M, Blaut M. 2003. Development of the intestinal bacterial composition in hospitalized preterm infants in comparison with breast-fed, full-term infants. Pediatric Research, Volume 54, Issue 3, Pages 393-399. PMID 12788986. Accessed September 3, 2007
- ↑ Mackie RI, Sghir A, Gaskins HR. 1999.Developmental microbial ecology of the neonatal gastrointestinal tract. American Journal of Clinical Nutrition, Volume 69, Issue 5, Pages 1035S-1045S. PMID 10232646. Accessed September 7, 2007
- ↑ Favier CF, Vaughan EE, De Vos WM, Akkermans AD. 2002. Molecular monitoring of succession of bacterial communities in human neonates. Applied and Environmental Microbiology, Volume 68, Issue 1, Pages 219-226. PMID 11772630.
- ↑ Coppa GV, Bruni S, Morelli L, Soldi S, Gabrielli O. 2004. The first prebiotics in humans: human milk oligosaccharides. Journal of Clinical Gastroenterology, Volume 38, Supplement 6, Pages S80-S83. PMID 15220665. Accessed September 3, 2007
- ↑ Harmsen HJ, Wildeboer-Veloo AC, Raangs GC, Wagendorp AA, Klijn N, Bindels JG, Welling GW. 2000. Analysis of intestinal flora development in breast-fed and formula-fed infants by using molecular identification and detection methods. Journal of Pediatric Gastroenterology and Nutrition, Volume 30, Issue 1, Pages 61-67. PMID 10630441. Accessed September 7, 2007
- ↑ Fanaro S, Chierici R, Guerrini P, Vigi V. 2003. Intestinal microflora in early infancy: composition and development. Acta Paediatrica, Volume 91, Issue 441, Pages 48-55. PMID 14599042. Accessed September 3, 2007
- ↑ 19.0 19.1 19.2 Wynne AG, McCartney AL, Brostoff J, Hudspith BN, Glenn GR and Gibson G. 2004. An in vitro assessment of the effects of broad-spectrum antibiotics on the human gut microflora and concomitant isolation of a Lactobacillus plantarum with anti-Candida activities. Anaerobe, Volume 10, Issue 3, Pages 165-169. PMID 16701514. Accessed September 3, 2007
- ↑ 20.0 20.1 20.2 20.3 Keeley J. 2004. Good bacteria trigger proteins to protect the gut. Howard Hughes Medical Institute. EurekAlert. Accessed January 9, 2007
- ↑ Jewell AP. 2005. Is the liver an important site for the development of immune tolerance to tumours? Medical Hypotheses, Volume 64, Issue 4, Pages 751-754. PMID 15694692. Accessed September 7, 2007
- ↑ 22.0 22.1 22.2 22.3 22.4 22.5 22.6 22.7 Guarner F and Malagelada JR. 2003. Role of bacteria in experimental colitis. Best Practice & Research Clinical Gastroenterology, Volume 17, Issue 5, October 2003, Pages 793-804. PMID 14507589. Accessed September 15, 2007
- ↑ 23.0 23.1 23.2 23.3 Carman RJ, Simon MA, Fernández H, Miller MA, and Bartholomew MJ. 2004. Ciprofloxacin at low levels disrupts colonization resistance of human fecal microflora growing in chemostats. Regulatory Toxicology and Pharmacology, Volume 40, Issue 3, December, Pages 319-326. PMID 15546686. Accessed September 7, 2007
- ↑ Knight DJW and Girling KJ. 2003. Gut flora in health and disease. The Lancet, Volume 361, Issue 9371, Page 1831. Accessed January 7, 2007
- ↑ 25.0 25.1 25.2 25.3 Suenaert P, Bulteel V, Lemmens L, Noman M, Geypens B, Assche GV, Geboes K, Ceuppens JL and Rutgeert P. 2002. Anti-tumor necrosis factor treatment restores the gut barrier in Crohn’s disease. The American Journal of Gastroenterology, Volume 97, Issue 8, Pages 2000-2004. PMID 12190167. Accessed September 7, 2007
- ↑ Garcia-Tsao G and Wiest R. 2004. Gut microflora in the pathogenesis of the complications of cirrhosis. Best Practice & Research Clinical Gastroenterology, Volume 18, Issue 2, Pages 353-372. PMID 15123075. Accessed September 7, 2007
- ↑ 27.0 27.1 27.2 Hugot JP. 2004. Inflammatory bowel disease: a complex group of genetic disorders. Best Practice & Research Clinical Gastroenterology, Volume 18, Issue 3, Pages 451-462. PMID 15157820. Accessed September 7, 2007
- ↑ 28.0 28.1 Veltkamp C, Tonkonogy SL, De Jong YP, Albright C, Grenther WB, Balish E, Terhorst C, and Sartor RB. 2001. Continuous stimulation by normal luminal bacteria is essential for the development and perpetuation of colitis in Tg(epsilon26) mice. Gastroenterology, Volume 120, Issue 4, Pages 900-913. PMID 11231944. Accessed September 7, 2007
- ↑ Ley RE, Turnbaugh PJ, Klein S, Gordon JI. Microbial ecology: human gut microbes associated with obesity. Nature, 2006 Volume 444, Issue 7122, Pages 1022-1023. PMID 17183309. Accessed September 7, 2007
- ↑ Turnbaugh PJ, Ley RE, Mahowald MA, Magrini V, Mardis ER, Gordon JI. 2006. An obesity-associated gut microbiome with increased capacity for energy harvest. Nature, Volume 444, Issue 7122, Pages 1027-1031. PMID 17183312. Accessed September 7, 2007
- ↑ Bäckhed F, Manchester JK, Semenkovich CF, Gordon JI. 2007. Mechanisms underlying the resistance to diet-induced obesity in germ-free mice. Proceedings of the National Academy of Sciences of the USA, Volume 104, Issue 3, Pages 979-984. PMID 17210919. Accessed September 7, 2007
- ↑ Bäckhed F, Ding H, Wang T, Hooper LV, Koh GY, Nagy A, Semenkovich CF, Gordon JI. The gut microbiota as an environmental factor that regulates fat storage. Proceedings of the National Academy of Sciences of the USA, Volume 101, Issue 44, Pages 15718-15723. PMID 15505215. Accessed September 7, 2007