Sickle-cell disease pathophysiology
https://https://www.youtube.com/watch?v=1ql-X60CUNQ%7C350}} |
Sickle-cell disease Microchapters |
Diagnosis |
---|
Treatment |
Case Studies |
Sickle-cell disease pathophysiology On the Web |
American Roentgen Ray Society Images of Sickle-cell disease pathophysiology |
Risk calculators and risk factors for Sickle-cell disease pathophysiology |
Editor-In-Chief: C. Michael Gibson, M.S., M.D. [1]; Associate Editor(s)-in-Chief: Aarti Narayan, M.B.B.S [2], Shyam Patel [3]
Overview
The pathophysiology of sickle cell disease is based on a mutation in the beta-globin chain of hemoglobin, which leads to red blood cell sickling and vaso-occlusive crises.
Pathophysiology
It is important to understand normal red blood cell physiology prior to understanding the pathophysiology of sickle cell disease. Normally, in healthy persons, red blood cells are deformable and flexible, allowing them to pass through vasculature.[1] Red blood cells lose their organelles and nucleus so they can easily pass through capillaries. They have a biconcave disc structure which allows for maximum oxygen transportation and deformability. Red blood cells can pass through capillaries that have 50% of the diameter than the red blood cells themselves.
Sickle-cell anemia is caused by a point mutation in the β-globin chain of hemoglobin, replacing the amino acid glutamic acid with the less polar amino acid valine at the sixth position of the β chain. The mutation occurs in exon 1 and changes the nucleic acid sequence from GAG to GTG.[2] Because valine is a hydrophobic amino acid, this imparts a sticky adhesive quality and results in sickling. Glutamic acid is a negatively charged amino acid and thus prevents red blood cells from sickling. The pathophysiology involves polymerization of deoxygenated HbS, which forms long fibers within erythrocytes and thus creates distortion of cell morphology.[3] The association of two wild-type α-globin subunits with two mutant β-globin subunits forms hemoglobin S, which polymerizes under low oxygen conditions causing distortion of red blood cells and a tendency for them to lose their elasticity.
New erythrocytes are quite elastic, which allows the cells to deform to pass through capillaries. Often a cycle occurs because as the cells sickle, they cause a region of low oxygen concentration which causes more red blood cells to sickle. Repeated episodes of sickling causes loss of this elasticity and the cells fail to return to normal shape when oxygen concentration increases. These rigid red blood cells are unable to flow through narrow capillaries, causing vessel occlusion and ischaemia.
Abnormal cell adhesion underlies the cellular pathophysiology of the disease.[1] When cells adhere to the vascular endothelium, obstruction can occur.[1] In addition to defective red blood cells, patients with sickle cell disease have abnormal white blood cells and platelets. These cell types are also more adhesive to the vascular endothelium. Adhesion in the post-capillary venules can result in venular obstruction.[4] Patients with sickle cell disease have a diminished vasodilatory response to nitric oxide.[4]
Vaso-occlusive crises
A vaso-occlusive crisis is caused by sickle-shaped red blood cells that obstruct capillaries and restrict blood flow to an organ, resulting in ischemia, pain, and organ damage.
Because of its narrow vessels and function in clearing defective red blood cells, the spleen is frequently affected. It is usually infarcted before the end of childhood in individuals suffering from sickle-cell anemia. This autosplenectomy increases the risk of infection from encapsulated organisms; preventive antibiotics and vaccinations are recommended for those with such asplenia.[5][6]
Bones, especially weight-bearing bones, are also a common target of vaso-occlusive damage. This is due to bone ischemia.
A recognized type of sickle crisis is the acute chest crisis, a condition characterized by fever, chest pain, labored breathing, and pulmonary infiltrate on chest x ray. Given that pneumonia and intrapulmonary sickling can both produce these symptoms, the patient is treated for both conditions.
Other sickle-cell crises
- Aplastic crises are acute deteriorations of the patient's baseline anemia producing pallor, tachycardia, and fatigue. This crisis is triggered by parvovirus B19, which directly affects erythropoiesis (production of red blood cells). Parvovirus infection nearly completely prevents red blood cell production for 2-3 days. In normal individuals, this is of little consequence, however, the shortened red cell life of sickle-cell patients results in an abrupt, life-threatening situation. Reticulocyte counts drop dramatically during the illness and the rapid turnover of red cells leads to the drop in hemoglobin. Most patients can be managed supportively; some need blood transfusion.
- Splenic sequestration crises are acute, painful enlargements of the spleen. The abdomen becomes bloated and very hard. Management is supportive, sometimes with blood transfusion.
Genetics
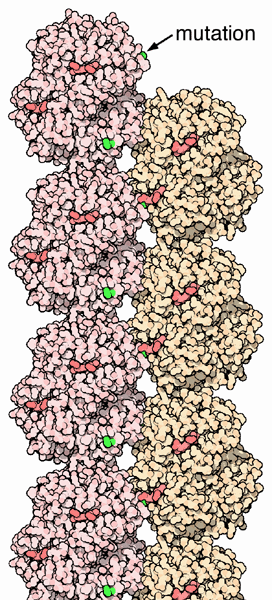
In people heterozygous for HgbS (carriers of sickling hemoglobin), the polymerization problems are minor. In people homozygous for HgbS, the presence of long chain polymers of HbS distort the shape of the red blood cell, from a smooth, donut-like shape to ragged and full of spikes, making it fragile and susceptible to breaking within capillaries. Carriers only have symptoms if they are deprived of oxygen (for example, while climbing a mountain) or while severely dehydrated. Normally these painful crises occur 0.8 times per year per patient. The sickle cell disease occurs when the sixth amino acid, glutamic acid is replaced by valine to change is structure and function.
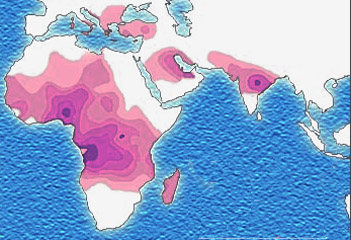
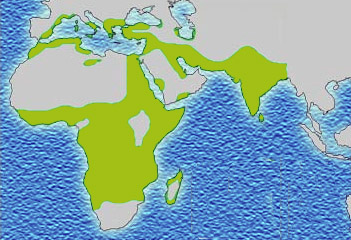
The gene defect is a known mutation of a single nucleotide (see single nucleotide polymorphism - SNP) (A to T) of the β-globin gene, which results in glutamic acid to be substituted by valine at position 6. Hemoglobin S with this mutation are referred to as HbS, as opposed to the normal adult HbA. The genetic disorder is due to the mutation of a single nucleotide, from a GAG to GUG codon mutation. This is normally a benign mutation, causing no apparent effects on the secondary, tertiary, or quaternary structure of hemoglobin. What it does allow for, under conditions of low oxygen concentration, is the polymerization of the HbS itself. The deoxygenated form of hemoglobin exposes a hydrophobic patch on the protein between the E and F helices. The hydrophobic residues of the valine at position 6 of the beta chain in hemoglobin are able to bind to the hydrophobic patch, causing hemoglobin S molecules to aggregate and form fibrous precipitates.
The allele responsible for sickle-cell anemia is autosomal recessive and can be found on the 11th chromosome. A person who receives the defective gene from both father and mother develops the disease; a person who receives one defective and one healthy allele remains healthy, but can pass on the disease and is known as a carrier. If two parents who are carriers have a child, there is a 25% chance of their child developing the illness and a 50% chance of their child just being a carrier. Since the gene is incompletely recessive, carriers have a few sickle red blood cells at all times, not enough to cause symptoms, but enough to give resistance to malaria. Because of this, heterozygotes have a higher fitness than either of the homozygotes. This is known as heterozygote advantage.
Due to the evolutionary advantage of the heterozygote, the illness is still prevalent, especially among people with recent ancestry in malaria-stricken areas, such as Africa, the Mediterranean, India, and the Middle East.[7]
The Price equation is a simplified mathematical model of the genetic evolution of sickle cell anemia.
The malaria parasite has a complex life cycle and spends part of it in red blood cells. In a carrier, the presence of the malaria parasite causes the red blood cell to rupture, making the plasmodium unable to reproduce. Further, the polymerization of Hb affects the ability of the parasite to digest Hb in the first place. Therefore, in areas where malaria is a problem, people's chances of survival actually increase if they carry sickle cell trait (selection for the heterozygote).
In the United States, where there is no endemic malaria, the incidence of sickle cell anemia amongst African Americans is lower (about 8%) than in West Africa and is falling. Without endemic malaria from Africa, the condition is purely disadvantageous, and will tend to be breed out of the affected population.
Pathology
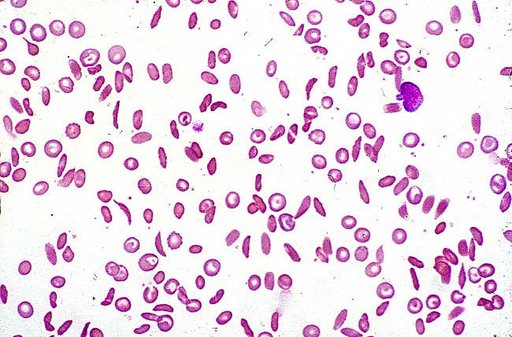
Inheritance
- Sickle-cell conditions are inherited from parents in much the same way as blood type, hair color and texture, eye color and other physical traits.
- The types of hemoglobin a person makes in the red blood cells depend upon what hemoglobin genes the person inherits from his or her parents.
Examples
- If one parent has sickle-cell anemia ("rr" in the diagram above) and the other is Normal (RR), all of their children will have sickle cell trait (Rr).
- If one parent has sickle-cell anemia (rr) and the other has Sickle Cell Trait (Rr), there is a 50% chance (or 1 out of 2) of a child having sickle cell disease (rr) and a 50% chance of a child having sickle cell trait (Rr).
- When both parents have Sickle Cell Trait (Rr), they have a 25% chance (1 of 4) of a child having sickle cell disease (rr), as shown in the diagram above.
- Sickle-cell anemia is caused by a recessive allele. Two carrier parents have a one in four chance of having a child with the disease. The child will be homozygous recessive.
References
- ↑ 1.0 1.1 1.2 Alapan Y, Kim C, Adhikari A, Gray KE, Gurkan-Cavusoglu E, Little JA; et al. (2016). "Sickle cell disease biochip: a functional red blood cell adhesion assay for monitoring sickle cell disease". Transl Res. 173: 74–91.e8. doi:10.1016/j.trsl.2016.03.008. PMC 4959913. PMID 27063958.
- ↑ Ballas SK, Kesen MR, Goldberg MF, Lutty GA, Dampier C, Osunkwo I; et al. (2012). "Beyond the definitions of the phenotypic complications of sickle cell disease: an update on management". ScientificWorldJournal. 2012: 949535. doi:10.1100/2012/949535. PMC 3415156. PMID 22924029.
- ↑ Colah RB, Mukherjee MB, Martin S, Ghosh K (2015). "Sickle cell disease in tribal populations in India". Indian J Med Res. 141 (5): 509–15. PMC 4510747. PMID 26139766.
- ↑ 4.0 4.1 Kato GJ (2012). "Priapism in sickle-cell disease: a hematologist's perspective". J Sex Med. 9 (1): 70–8. doi:10.1111/j.1743-6109.2011.02287.x. PMC 3253142. PMID 21554552.
- ↑ Pearson H. "Sickle cell anemia and severe infections due to encapsulated bacteria". J Infect Dis. 136 Suppl: S25–30. PMID 330779.
- ↑ Wong W, Powars D, Chan L, Hiti A, Johnson C, Overturf G (1992). "Polysaccharide encapsulated bacterial infection in sickle cell anemia: a thirty year epidemiologic experience". Am J Hematol. 39 (3): 176–82. PMID 1546714.
- ↑ Kwiatkowski, DP (2005). "How Malaria Has Affected the Human Genome and What Human Genetics Can Teach Us about Malaria". Am J Hum Genet. 77: 171–92. PMID 16001361. External link in
|title=
(help) - ↑ http://picasaweb.google.com/mcmumbi/USMLEIIImages