Glycosaminoglycan
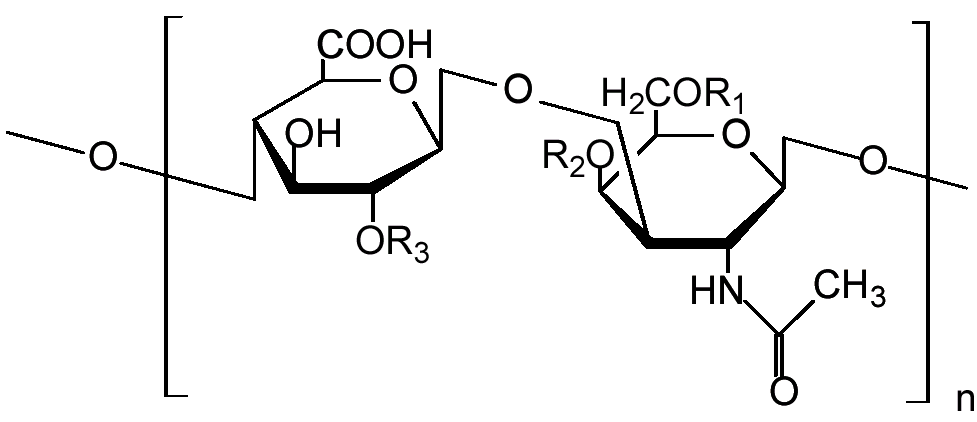

WikiDoc Resources for Glycosaminoglycan |
Articles |
---|
Most recent articles on Glycosaminoglycan Most cited articles on Glycosaminoglycan |
Media |
Powerpoint slides on Glycosaminoglycan |
Evidence Based Medicine |
Cochrane Collaboration on Glycosaminoglycan |
Clinical Trials |
Ongoing Trials on Glycosaminoglycan at Clinical Trials.gov Trial results on Glycosaminoglycan Clinical Trials on Glycosaminoglycan at Google
|
Guidelines / Policies / Govt |
US National Guidelines Clearinghouse on Glycosaminoglycan NICE Guidance on Glycosaminoglycan
|
Books |
News |
Commentary |
Definitions |
Patient Resources / Community |
Patient resources on Glycosaminoglycan Discussion groups on Glycosaminoglycan Patient Handouts on Glycosaminoglycan Directions to Hospitals Treating Glycosaminoglycan Risk calculators and risk factors for Glycosaminoglycan
|
Healthcare Provider Resources |
Causes & Risk Factors for Glycosaminoglycan |
Continuing Medical Education (CME) |
International |
|
Business |
Experimental / Informatics |
Editor-In-Chief: C. Michael Gibson, M.S., M.D. [1]
Overview
Glycosaminoglycans[1] (GAGs) or mucopolysaccharides[2] are long unbranched polysaccharides consisting of a repeating disaccharide unit. The repeating unit (except for keratan) consists of an amino sugar (N-acetylglucosamine or N-acetylgalactosamine) along with a uronic sugar (glucuronic acid or iduronic acid) or galactose.[3] Glycosaminoglycans are highly polar and attract water. They are therefore useful to the body as a lubricant or as a shock absorber.
Production
Glycosaminoglycans have high degrees of heterogeneity with regards to molecular mass, disaccharide construction, and sulfation due to the fact that GAG synthesis, unlike proteins or nucleic acids, is not template driven, and dynamically modulated by processing enzymes.[4]
Based on core disaccharide structures, GAGs are classified into four groups.[5] Heparin/heparan sulfate (HSGAGs) and chondroitin sulfate/dermatan sulfate (CSGAGs) are synthesized in the Golgi apparatus, where protein cores made in the rough endoplasmic reticulum are posttranslationally modified with O-linked glycosylations by glycosyltransferases forming proteoglycans. Keratan sulfate may modify core proteins through N-linked glycosylation or O-linked glycosylation of the proteoglycan. The fourth class of GAG, hyaluronic acid, is not synthesized by the Golgi, but rather by integral membrane synthases which immediately secrete the dynamically elongated disaccharide chain.
HSGAG and CSGAG modified proteoglycans first begin with a consensus Ser-Gly/Ala-X-Gly motif in the core protein. Construction of a tetrasaccharide linker that consists of -GlcAβ1–3Galβ1–3Galβ1–4Xylβ1-O-(Ser)-, where xylosyltransferase, β4-galactosyl transferase (GalTI),β3-galactosyl transferase (GalT-II), and β3-GlcA transferase (GlcAT-I) transfer the four monosaccharides, begins synthesis of the GAG modified protein. The first modification of the tetrasaccharide linker determines whether the HSGAGs or CSGAGs will be added. Addition of a GlcNAc promotes the addition of HSGAGs while addition of GalNAc to the tetrasaccharide linker promotoes CSGAG development.[5] GlcNAcT-I transfers GlcNAc to the tetrasaccahride linker, which is distinct from glycosyltransferase GlcNAcT-II, the enzyme that is utilized to build HSGAGs. Interestingly, EXTL2 and EXTL3, two genes in the EXT tumor suppressor family, have been shown to have GlcNAcT-I activity. Conversely, GalNAc is transferred to the linker by the enzyme GalNAcT to initiate synthesis of CSGAGs, an enzyme which may or may not have distinct activity compared to the GalNAc transferase activity of chondroitin synthase.[5]
With regards to HSGAGs, a multimeric enzyme encoded by EXT1 and EXT2 of the EXT family of genes, transfers both GlcNAc and GlcA for HSGAG chain elongation. While elongating, the HSGAG is dynamically modified, first by N-deacetylase, N-sulfotransferase (NDST1), which is a bifunctional enyzme that cleaves the N-acetyl group from GlcNAc and subsequently sulfates the N-position. Next, C-5 uronyl epimerase coverts d-GlcA to l-IdoA followed by 2-O sulfation of the uronic acid sugar by 2-O sulfotransferase (Heparan sulfate 2-O-sulfotransferase). Finally, the 6-O and 3-O positions of GlcNAc moities are sulfated by 6-O (Heparan sulfate 6-O-sulfotransferase) and 3-O (3-OST) sulfotransferases.
Chondroitin sulfate and dermatan sulfate, which comprise CSGAGs, are differentiated from each other by the presence of GlcA and IdoA epimers respectively. Similar to the production of HSGAGs, C-5 uronyl epimerase converts d-GlcA to l-IdoA to synthesize dermatan sulfate. Three sulfation events of the CSGAG chains occur: 4-O and/or 6-O sulfation of GalNAc and 2-O sulfation of uronic acid. Four isoforms of the 4-O GalNAc sulfotransferases (C4ST-1, C4ST-2, C4ST-3, and D4ST-1) and three isoforms of the GalNAc 6-O sulfotransferases (C6ST, C6ST-2, and GalNAc4S-6ST) are responsible for the sulfation of GalNAc.[6]
Unlike HSGAGs and CSGAGs, the third class of GAGs, those belonging to keratan sulfate types, are driven towards biosynthesis through particular protein sequence motifs. For example, in the cornea and cartilage, the keratan sulfate domain of aggrecan consists of a series of tandemly repeated hexapeptides with a consensus sequence of E(E/L)PFPS.[7] Additionally, for three other keratan sulfated proteoglycans, lumican, keratocan, and mimecan (OGN), the consensus sequence NX(T/S) along with protein secondary structure was determined to be involved in N-linked oligosaccharide extension with keratan sulfate.[7] Keratan sulfate elongation begins at the nonreducing ends of three linkage oligosaccharides, which define the three classes of keratan sulfate. Keratan sulfate I (KSI) is N -linked via a high mannose type precursor oligosaccharide. Keratan sulfate II (KSII) and keratan sulfate III (KSIII) are O-linked, with KSII linkages identical to that of mucin core structure, and KSIII linked to a 2-O mannose. Elongation of the keratan sulfate polymer occurs through the glycosyltransferase addition of Gal and GlcNAc. Galactose addition occurs primarily through the β-1,4-galactosyltransferase enzyme (β4Gal-T1) while the enzymes responsible for β-3-Nacetylglucosamine have not been clearly identified. Finally, sulfation of the polymer occurs at the 6-position of both sugar residues. The enzyme KS-Gal6ST (CHST1) transfers sulfate groups to galactose while N-acetylglucosaminyl-6-sulfotransferase (GlcNAc6ST) (CHST2) transfers sulfate groups to terminal GlcNAc in keratan sulfate.[8]
The fourth class of GAG, hyaluronic acid, is synthesized by three transmembrane synthase proteins HAS1, HAS2, and HAS3. HA, a linear polysaccharide, is composed of repeating disaccharide units of →4)GlcAβ(1→3)GlcNAcβ(1→ and has a very high molecular mass, ranging from 105 to 107 Da. Each HAS enzyme is capable of transglycosylation when supplied with UDP-GlcA and UDP-GlcNAc.[9][10] HAS2 is responsible for very large hyaluronic acid polymers, while smaller sizes of HA are synthesized by HAS1 and HAS3. While each HAS isoform catalyzes the same biosynthetic reaction, each HAS isoform is independently active. HAS isoforms have also been shown to have differing Km values for UDP-GlcA and UDPGlcNAc.[11] It is believed that through differences in enzyme activity and expression, the wide spectrum of biological functions mediated by HA can be regulated.
Function
Endogenous heparin is localized and stored in secretory granules of mast cells. Histamine that is present within the granules is protonated (H2A2+) at pH within granules (5.2-6.0), thus it is believed that heparin, which is highly negatively charged, functions to electrostatically retain and store histamine.[12] In the clinic, heparin is administered as an anticoagulant and is also the first line choice for thromboembolic diseases.[13][14] Heparan sulfate (HS) has numerous biological activities and functions, including cell adhesion, regulation of cell growth and proliferation, developmental processes, cell surface binding of lipoprotein lipase and other proteins, angiogenesis, viral invasion, and tumor metastasis.[12]
CSGAGs interact with heparin binding proteins, specifically dermatan sulfate interactions with fibroblast growth factor FGF-2 and FGF-7 have been implicated in cellular proliferation and wound repair [15] while interactions with hepatic growth factor/scatter factor (HGF/SF) activate the HGF/SF signaling pathway (c-Met) through its receptor. Other biological functions for which CSGAGs are known to play critical functions in include inhibition of axonal growth and regeneration in CNS development, roles in brain development, neuritogenic activity, and pathogen infection.[16]
One of the main functions of the third class of GAGs, keratan sulfates, is the maintenance of tissue hydration. Within the normal cornea, dermatan sulfate is fully hydrated whereas keratan sulfate is only partially hydrated suggesting that keratan sulfate may behave as a dynamically controlled buffer for hydration.[17] In disease states such as macular corneal dystrophy, in which GAGs levels such as KS are altered, loss of hydration within the corneal stroma is believed to be the cause of corneal haze, thus supporting the long held hypothesis that corneal transparency is a dependent on proper levels of keratan sulfate. Keratan sulfate GAGs are found in many other tissues besides the cornea, where they are known to regulate macrophage adhesion, form barriers to neurite growth, regulate embryo implantation in the endometrial uterine lining during menstrual cycles, and affect the motility of corneal endothelial cells.[17] In summary, KS plays an anti-adhesive role, which suggests very important functions of KS in cell motility and attachment as well as other potential biological processes.
Hyaluronic acid is a major component of synovial tissues and fluid, as well as other soft tissues, and endows their environments with remarkable rheological properties. For example, solutions of hyaluronic acid are known to be viscoelastic, and viscosity changes with shear stress. At low shear stress, a solution of 10 g/L of hyaluronic acid may have a viscosity 106 times the viscosity of the solvent, while under high shear stress, viscosity may drop by as much as 103 times.[18] The aforementioned rheological properties of solutions of hyaluronic acid make it ideal for lubricating joints and surfaces that move along each other, such as cartilage. In vivo, hyaluronic acid forms hydrated coils that form randomly kinked coils that entangle to form a network. Hyaluronan networks retard diffusion and form a diffusion barrier that regulates transport of substances through intercellular spaces. For example, hyaluronan takes part in the partitioning of plasma proteins between vascular and extravascular spaces, and it is this excluded volume phenomenon that affects solubility of macromolecules in the interstitium, changes chemical equilibria, and stabilizes the structure of collagen fibers.[18] Other functions include matrix interactions with hyaluronan binding proteins such as hyaluronectin, glial hyaluronan binding protein, brain enriched hyaluronan binding protein, collagen VI, TSG-6, and inter-alpha-trypsin inhibitor. Cell surface interactions involving hyaluronan are its well-known coupling with CD44, which may be related to tumor progression, and also with RHAMM (Hyaluronan-mediated motility receptor), which has been implicated in developmental processes, tumor metastasis, and pathological reparative processes. Fibroblasts, mesothelial cells, and certain types of stem cells surround themselves in a pericellular "coat", part of which is constructed from hyaluronan, in order to shield themselves from bacteria, red blood cells, or other matrix molecules. For example, with regards to stem cells, hyaluronan, along with chondroitin sulfate, helps to form the stem cell niche. Stem cells are protected from the effects of growth factors by a shield of hyaluronan and minimally sulfated chondroitin sulfate. During progenitor division, the daughter cell moves outside of this pericellular shield where it can then be influenced by growth factors to differentiate even further.
Classification
Members of the glycosaminoglycan family vary in the type of hexosamine, hexose or hexuronic acid unit they contain (e.g. glucuronic acid, iduronic acid, galactose, galactosamine, glucosamine).
They also vary in the geometry of the glycosidic linkage.
Examples of GAGs include:
Name | Hexuronic acid / Hexose | Hexosamine | Linkage geometry between predominant monomeric units | Unique features |
Chondroitin sulfate | GlcUA or GlcUA(2S) | GalNAc or GalNAc(4S) or GalNAc(6S) or GalNAc(4S,6S) | 'GlcUAβ1-3'GalNAcβ1-4 | Most prevalent GAG |
Dermatan sulfate | GlcUA or IdoUA or IdoUA(2S) | GalNAc or GalNAc(4S) or GalNAc(6S) or GalNAc(4S,6S) | 'IdoUAβ1-3'GalNAcβ1-4 | Distinguished from chondroitin sulfate by the presence of iduronic acid, although some hexuronic acid monosaccharides may be glucuronic acid.[15] |
Keratan sulfate | Gal or Gal(6S) | GlcNAc or GlcNAc(6S) | -Gal(6S)β1-4GlcNAc(6S)β1-3 | Keratan sulfate type II may be fucosylated.[19] |
Heparin | GlcUA or IdoUA(2S) | GlcNAc or GlcNS or GlcNAc(6S) or GlcNS(6S) | -IdoUA(2S)α1-4GlcNS(6S)α1-4 | Highest negative charge density of any known biological molecule |
Heparan sulfate | GlcUA or IdoUA or IdoUA(2S) | GlcNAc or GlcNS or GlcNAc(6S) or GlcNS(6S) | -GlcUAβ1-4GlcNAcα1-4 | Highly similar in structure to heparin, however heparan sulfate's disaccharide units are organised into distinct sulfated and non-sulfated domains.[20] |
Hyaluronan | GlcUA | GlcNAc | -GlcUAβ1-3GlcNAcβ1-4 | The only GAG that is exclusively non-sulfated |
Abbreviations
- GlcUA = β-D-glucuronic acid
- GlcUA(2S) = 2-O-sulfo-β-D-glucuronic acid
- IdoUA = α-L-iduronic acid
- IdoUA(2S) = 2-O-sulfo-α-L-iduronic acid
- Gal = β-D-galactose
- Gal(6S) = 6-O-sulfo-β-D-galactose
- GalNAc = β-D-N-acetylgalactosamine
- GalNAc(4S) = β-D-N-acetylgalactosamine-4-O-sulfate
- GalNAc(6S) = β-D-N-acetylgalactosamine-6-O-sulfate
- GalNAc(4S,6S) = β-D-N-acetylgalactosamine-4-O, 6-O-sulfate
- GlcNAc = α-D-N-acetylglucosamine
- GlcNS = α-D-N-sulfoglucosamine
- GlcNS(6S) = α-D-N-sulfoglucosamine-6-O-sulfate
See also
- Mucopolysaccharidosis (lysosomal storage diseases)
- Lipopolysaccharide
References
- ↑ Template:DorlandsDict
- ↑ Template:DorlandsDict
- ↑ Esko, Jeffrey D; Kimata, Koji; Lindahl, Ulf (2009). "Chapter 16: Proteoglycans and Sulfated Glycosaminoglycans". Essentials of Glycobiology. Cold Spring Harbor Laboratory Press. ISBN 0879695595.
- ↑ Caligur, Vicki (2008). "Glycosaminoglycan Sulfation and Signaling". Retrieved 25 November 2012.
- ↑ 5.0 5.1 5.2 Sasisekharan, Ram; Raman, Rahul; Prabhakar, Vikas (August 2006). "GLYCOMICS APPROACH TO STRUCTURE-FUNCTION RELATIONSHIPS OF GLYCOSAMINOGLYCANS". Annual Review of Biomedical Engineering. 8 (1): 181–231. doi:10.1146/annurev.bioeng.8.061505.095745. Retrieved 12 December 2014.
- ↑ Kusche-Gullberg M, Kjellén L. (2003). "Sulfotransferases in glycosaminoglycan biosynthesis". Current Opinion in Structural Biology. 13 (5): 605–11. doi:10.1016/j.sbi.2003.08.002. PMID 14568616.
- ↑ 7.0 7.1 Funderburgh JL. (2002). "Keratan sulfate biosynthesis". IUBMB Life. 54 (4): 187–94. doi:10.1080/15216540214932. PMC 2874674. PMID 12512857.
- ↑ Yamamoto Y, Takahashi I, Ogata N, Nakazawa K. (2001). "Purification and characterization of N-acetylglucosaminyl sulfotransferase from chick corneas". Archives of Biochemistry and Biophysics. 392 (1): 87–92. doi:10.1006/abbi.2001.2422. PMID 11469798.
- ↑ Yoshida M, Itano N, Yamada Y, Kimata K. (2000). "In vitro synthesis of hyaluronan by a single protein derived from mouse HAS1 gene and characterization of amino acid residues essential for the activity". The Journal of Biological Chemistry. 275 (1): 497–506. doi:10.1074/jbc.275.1.497. PMID 10617644.
- ↑ DeAngelis PL, and Weigel PH (1994). "Immunochemical confirmation of the primary structure of streptococcal hyaluronan synthase and synthesis of high molecular weight product by the recombinant enzyme". Biochemistry. 33 (31): 9033–9039. doi:10.1021/bi00197a001. PMID 8049203.
- ↑ Itano N, Sawai T, Yoshida M, Lenas P, Yamada Y, Imagawa M, Shinomura T, Hamaguchi M., Yoshida Y, Ohnuki Y, Miyauchi S, Spicer AP, McDonald JA, and Kimata K. (1999). "Three isoforms of mammalian hyaluronan synthases have distinct enzymatic properties". Journal of Biological Chemistry. 274 (35): 25085–92. doi:10.1074/jbc.274.35.25085. PMID 10455188.
- ↑ 12.0 12.1 Rabenstein DL. (2002). "Heparin and heparan sulfate: structure and function". Natural Products Reports. 19: 312–331. doi:10.1039/B100916H. PMID 12137280.
- ↑ Jin L, Abrahams JP, Skinner R, Petitou M, Pike RN, Carrell RW. (1997). "The anticoagulant activation of antithrombin by heparin". Proceedings of the National Academy of Sciences of the United States of America. 94 (26): 14683–8. doi:10.1073/pnas.94.26.14683. PMC 25092. PMID 9405673.
- ↑ Rodén, L. (1989). Lane, DA, ed. Heparin: Chemical and Biological Properties, ClinicalApplications. CRC Press, Inc. p. 1.
- ↑ 15.0 15.1 Trowbridge JM, Gallo RL. (2002). "Dermatan sulfate: new functions from an old glycosaminoglycan". Glycobiology. 12 (9): 117R–125R. doi:10.1093/glycob/cwf066. PMID 12213784.
- ↑ Sugahara K, Mikami T, Uyama T, Mizuguchi S, Nomura K, Kitagawa H. (2003). "Recent advances in the structural biology of chondroitin sulfate and dermatan sulfate". Current Opinion in Structural Biology. 13 (5): 612–620. doi:10.1016/j.sbi.2003.09.011. PMID 14568617.
- ↑ 17.0 17.1 Funderburgh, JL. (2000). "Keratan sulfate: structure, biosynthesis, and function". Glycobiology. 10 (10): 951–8. doi:10.1093/glycob/10.10.951. PMID 11030741.
- ↑ 18.0 18.1 Laurent TC, Laurent UB, Fraser JR. (1996). "The structure and function of hyaluronan: An overview". Immunology and Cell Biology. 74 (2): A1–7. doi:10.1038/icb.1996.32. PMID 8724014.
- ↑ Funderburgh JL. (2000). "Keratan sulfate: structure, biosynthesis, and function". Glycobiology. 10 (10): 951–958. doi:10.1093/glycob/10.10.951. PMID 11030741.
- ↑ Gallagher, J.T., Lyon, M. (2000). "Molecular structure of Heparan Sulfate and interactions with growth factors and morphogens". In Iozzo, M, V. Proteoglycans: structure, biology and molecular interactions. Marcel Dekker Inc. New York, New York. pp. 27–59. ISBN 978-0-8247-0334-9.
External links
- King M. 2005. Glycosaminoglycans. Indiana University School of Medicine Accessed December 31, 2006.
- Glycosaminoglycans at the US National Library of Medicine Medical Subject Headings (MeSH)
- MRI evaluation of glycosaminoglycan loss (dGEMRIC evaluation)