Color radiation astronomy
Editor-In-Chief: Henry A. Hoff
Each form of radiation that an astronomical entity can emit, absorb, fluoresce, transmit, or reflect has a spectrum in time, space, intensity, or variation.
A spectrum of variation shows its colors.
Astronomy
Astronomy in general consists of observing the types of spectra apparently originating with an astronomical entity and describing these observations empirically if necessary with theory.
Radiation
Radiation in its spectrum of forms from meteors to superluminals shows variation, time, space, and intensity.
Minerals
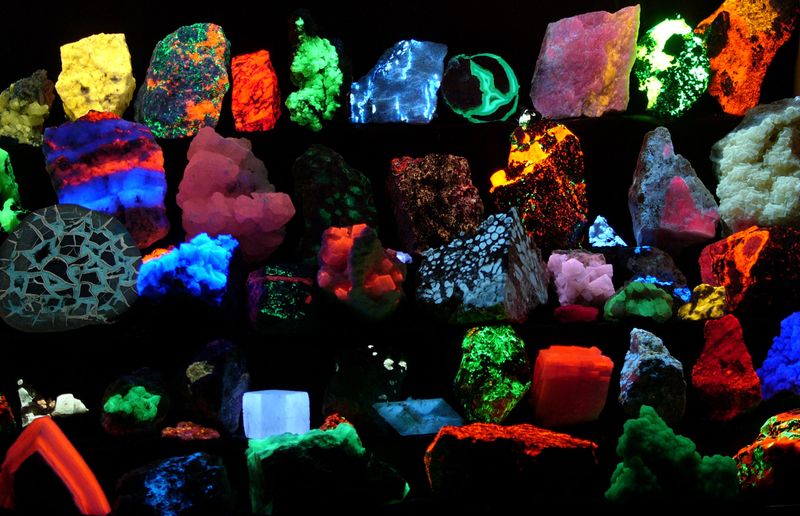
Fluorescence is the emission of light by a substance that has absorbed light or other electromagnetic radiation. It is a form of luminescence. In most cases, the emitted light has a longer wavelength, and therefore lower energy, than the absorbed radiation. However, when the absorbed electromagnetic radiation is intense, it is possible for one electron to absorb two photons; this two-photon absorption can lead to emission of radiation having a shorter wavelength than the absorbed radiation. The emitted radiation may also be of the same wavelength as the absorbed radiation, termed "resonance fluorescence".[1]
The most striking examples of fluorescence occur when the absorbed radiation is in the ultraviolet region of the spectrum, and thus invisible to the human eye, and the emitted light is in the visible region.
The common fluorescent lamp relies on fluorescence. Inside the glass tube is a partial vacuum and a small amount of mercury. An electric discharge in the tube causes the mercury atoms to emit ultraviolet light. The tube is lined with a coating of a fluorescent material, called the phosphor, which absorbs the ultraviolet and re-emits visible light. Fluorescent lighting is more energy-efficient than incandescent lighting elements. However, the uneven spectrum of traditional fluorescent lamps may cause certain colors to appear different than when illuminated by incandescent light or daylight. The mercury vapor emission spectrum is dominated by a short-wave UV line at 254 nm (which provides most of the energy to the phosphors), accompanied by visible light emission at 436 nm (blue), 546 nm (green) and 579 nm (yellow-orange). These three lines can be observed superimposed on the white continuum using a hand spectroscope, for light emitted by the usual white fluorescent tubes. These same visible lines, accompanied by the emission lines of trivalent europium and trivalent terbium, and further accompanied by the emission continuum of divalent europium in the blue region, comprise the more discontinuous light emission of the modern trichromatic phosphor systems used in many compact fluorescent lamp and traditional lamps where better color rendition is a goal.[2]
Ultraviolet lamps are also used in analyzing minerals and gems. Materials may look the same under visible light, but fluoresce to different degrees under ultraviolet light, or may fluoresce differently under short wave ultraviolet versus long wave ultraviolet.
Ultraviolet lamps may cause certain minerals to fluoresce, and is a key tool in prospecting for tungsten mineralisation.
Many samples of fluorite exhibit fluorescence under ultraviolet light, a property that takes its name from fluorite.[3] Many minerals, as well as other substances, fluoresce. Fluorescence involves the elevation of electron energy levels by quanta of ultraviolet light, followed by the progressive falling back of the electrons into their previous energy state, releasing quanta of visible light in the process. In fluorite, the visible light emitted is most commonly blue, but red, purple, yellow, green and white also occur. The fluorescence of fluorite may be due to mineral impurities such as yttrium, ytterbium, or organic matter in the crystal lattice. In particular, the blue fluorescence seen in fluorites from certain parts of Great Britain responsible for the naming of the phenomenon of fluorescence itself, has been attributed to the presence of inclusions of divalent europium in the crystal.[4]
"Between 190 and 1700 nm, the ordinary refractive index varies roughly between 1.9 and 1.5, while the extraordinary refractive index varies between 1.6 and 1.4.[5]
Under longwave (365 nm) ultraviolet light, diamond may fluoresce a blue, yellow, green, mauve, or red of varying intensity. The most common fluorescence is blue, and such stones may also phosphoresce yellow—this is thought to be a unique combination among gemstones. There is usually little if any response to shortwave ultraviolet.
Meteors
A spectrum of meteors may range in size (space), speed (time), numbers (intensity), or state (variation, e.g. rocky, liquid, gaseous, or plasma).
The 1910 approach, which came into naked-eye view around 10 April[6] and came to perihelion on 20 April,[6] was notable for several reasons: it was the first approach of which photographs exist, and the first for which spectroscopic data were obtained.[7] Furthermore, the comet made a relatively close approach of 0.15AU,[6] making it a spectacular sight. Indeed, on 19 May, the Earth actually passed through the tail of the comet.[8][9] One of the substances discovered in the tail by spectroscopic analysis was the toxic gas cyanogen,[10] which led astronomer Camille Flammarion to claim that, when Earth passed through the tail, the gas "would impregnate the atmosphere and possibly snuff out all life on the planet."[11] His pronouncement led to panicked buying of gas masks and quack "anti-comet pills" and "anti-comet umbrellas" by the public.[12] In reality, as other astronomers were quick to point out, the gas is so diffuse that the world suffered no ill effects from the passage through the tail.[11]
"It is quite possible that [faint streamers preceding the main tail and lying nearly in the prolonged radius vector] may have touched the Earth, probably between May 19.0 and May 19.5, [1910,] but the Earth must have passed considerably to the south of the main portion of the tail [of Halley's comet]."[13]
A typical comet nucleus has an albedo of 0.04.[14]
At left is an image of Comet West. "Comet West was a stunning sight in the predawn sky of March, 1976, bright with a tall and broad dust tail. ... [T]he comet [was] discovered on photographs taken in August 1975 by Richard West of the European Southern Observatory ... Comet West passed perihelion on February 25, 1976, at a distance of 0.20 a.u. [and] had reached about magnitude -3 at perihelion. Several observers saw it telescopically in daylight, and John Bortle observed it with the naked eye shortly before sunset. ... The following morning, March 7, ... It was brilliant, with a head as bright as Vega (which was nearly overhead) and a huge tail, about 20 degrees tall, straight near the bottom and bending to the left in its upper reaches. The comet quickly faded during March".[15]
Although many comets are photographed in black and white, not that many are actually only white but have colors. The image at right of McNaught Comet shows white and other colors, as does Comet West at left.
Of the Local Group, “[i]ts two dominant galaxies, the Milky Way and Andromeda (M31), are separated by a distance of ~700 kpc and are moving toward each other with a radial velocity of about -117 km s-1 (Binney & Tremaine 1987, p. 605).”[16] making Andromeda one of the few blueshifted galaxies. The Andromeda Galaxy and the Milky Way are thus expected to collide in about 4.5 billion years, although the details are uncertain since Andromeda's tangential velocity with respect to the Milky Way is only known to within about a factor of two.[17] A likely outcome of the collision is that the galaxies will merge to form a giant elliptical galaxy.[18] Such events are frequent among the galaxies in galaxy groups. The fate of the Earth and the Solar System in the event of a collision are currently unknown. If the galaxies do not merge, there is a small chance that the Solar System could be ejected from the Milky Way or join Andromeda.[19]
Asteroids
{{free media}}
The center image is a log-log plot of frequency vs. size for asteroids in the solar system.
Aerometeors
{{fairuse}}
"Several times a year an atmospheric river [shown in the image on the right forming over Hawai'i]—a long, narrow conveyor belt of storms that stream in relentlessly from the Pacific Ocean—drops inches of rain or feet of snow on the U.S. west coast. Such a system triggered floods and mudslides in central and southern California this past weekend [2-3 February 2019]."[20]
"Atmospheric rivers flow through the sky about a mile above the ocean surface, and may extend across a thousand miles of ocean to the coast. Some bring routine rain but the more intense systems can carry as much water as 15 Mississippi Rivers. The series of storms striking land can arrive for days or, occasionally, weeks on end. They hit west-facing coastlines worldwide, although the U.S. experiences more than most other national coasts."[20]
The “atmospheric river scale” "ranks severity and impacts, from category 1 (weak) to category 5 (exceptional)."[20]
"Without a scale, we really had no way to objectively communicate what would be a strong storm or a weak one."[21]
"Scientists, the media and the public viewed atmospheric rivers as primarily a hazard, but the weaker ARs are quite beneficial. Water managers made it clear to us that a rating scale would be helpful."[21]
"The scale, published Tuesday in the Bulletin of the American Meteorological Society, ranks atmospheric rivers on five levels:"[20]
- Category 1: Weak—primarily beneficial
- Category 2: Moderate—mostly beneficial, but also somewhat hazardous
- Category 3: Strong—balance of beneficial and hazardous
- Category 4: Extreme—mostly hazardous, but also beneficial (if persistent drought)
- Category 5—Exceptional—primarily hazardous
Cosmic rays
At right is an image indicating the range of cosmic-ray energies. The flux for the lowest energies (yellow zone) is mainly attributed to solar cosmic rays, intermediate energies (blue) to galactic cosmic rays, and highest energies (purple) to extragalactic cosmic rays.[22]
Cosmic rays may be upwards of a ZeV (1021 eV).
About 89% of cosmic rays are simple protons or hydrogen nuclei, 10% are helium nuclei of alpha particles, and 1% are the nuclei of heavier elements. Solitary electrons constitute much of the remaining 1%.
Def. cosmic rays that are created when primary cosmic rays interact with interstellar matter are called secondary cosmic rays.
Galactic cosmic rays
{{fairuse}}
{{free media}}
The "effect of time-variations in galactic cosmic rays on the rate of production of neutrons in the atmosphere [was studied using] a series of balloon and airplane observations of the [fast neutron] flux and spectrum of 1-10 MeV neutrons, in flights at high geomagnetic latitude, during [quiet times as well as during Forbush decreases, which are rapid decreases in the observed galactic cosmic rays following a coronal mass ejection (CME), and solar particle events for] the period of increasing solar modulation, 1965-1969. It also included latitude surveys in 1964-1965 and in 1968."[23]
In the image on the right for Forbush decreases, data include GOES-15 X-rays, energetic particles, and magnetometer. Cosmic Rays from the Moscow station show a Forbush Decrease.
The graph on the right shows an inverse correlation between sunspot numbers (solar activity) and neutron production from galactic cosmic rays.
Notation: let the symbol Z stand for atomic number.
- let the symbol PeV stand for 1015 electron volts.
"The most dominant group is the iron group (Z = 25 − 27), at energies around 70 PeV more than 50% of the all-particle flux consists of these elements."[24]
In the graph on the right, the black line is cosmic-ray data and the red line is temperature. Ulysses data is included.
Solar cosmic rays
{{fairuse}}
"A persistent problem of solar cosmic-ray research has been the lack of observations bearing on the timing and conditions in which protons that escape to the interplanetary medium are first accelerated in the corona."[25]
For solar cosmic-rays, the apparent lack of proton acceleration in the corona seems justified, in contrast to the electrons, proton bremsstrahlung and gyrosynchrotron emission are negligible. This suggests a transit time anomaly, ΔTA, defined as follows:
- ΔTA = ΔTonset - 11 min,
where ΔTonset is the deduced Sun-Earth transit time for the first arriving relativistic protons and 11 min is the nominal transit time for a ~2 GeV proton traversing a 1.3 AU Archimedes spiral path."[25] "The solar wind is a stream of charged particles ejected from the upper atmosphere of the Sun. It mostly consists of electrons and protons with energies usually between 1.5 and 10 keV.
ΔTA may have values from "7-19 min for a small sample of well-connected ... cosmic-ray flares."[25] The transit time anomaly may be explained by a rise time associated with the ground-level events (GLEs). "The average GLE rise time ... for well-connected ... events ... defined to be the time from event onset to maximum as measured by the neutron monitor station showing the largest increase and whose asymptotic cone of acceptance ... includes the nominal direction of the Archimedean spiral path, is 21.3 min."[25]
"Data from an extensive air shower detector of ultrahigh-energy cosmic rays shows shadowing of the cosmic-ray flux by the Moon and the Sun with significance of 4.9 standard deviations. This is the first observation of such shadowing."[26]
"The ... solar proton flare on 20 April 1998 at W 90° and S 43° (9:38 UT) was measured by the GOES-9-satellite (Solar Geophysical Data 1998), as well as by other experiments on WIND ... and GEOTAIL. Protons were accelerated up to energies > 110 MeV and are therefore able to hit the surface of Mercury."[27]
Anomalous cosmic rays
{{fairuse}}
"While interstellar plasma is kept outside the heliosphere by an interplanetary magnetic field, the interstellar neutral gas flows through the solar system like an interstellar wind, at a speed of 25 km/sec. When closer to the Sun, these atoms undergo the loss of one electron in photo-ionization or by charge exchange. Photo-ionization is when an electron is knocked off by a solar ultra-violet photon, and charge exchange involves giving up an electron to an ionized solar wind atom. Once these particles are charged, the Sun's magnetic field picks them up and carries them outward to the solar wind termination shock. They are called pickup ions during this part of their trip."[28]
"The ions repeatedly collide with the termination shock, gaining energy in the process. This continues until they escape from the shock and diffuse toward the inner heliosphere. Those that are accelerated are then known as anomalous cosmic rays."[28]
"ACRs [may] represent a sample of the very local interstellar medium. They are not thought to have experienced such violent processes as GCRs, and they have a lower speed and energy. ACRs include large quantities of helium, oxygen, neon, and other elements with high ionization potentials, that is, they require a great deal of energy to ionize, or form ions. ACRs are a tool for studying the movement of energetic particles within the solar system, for learning the general properties of the heliosphere, and for studying the nature of interstellar material itself."[28]
Neutrons
The neutron detection temperature, also called the neutron energy, indicates a free neutron's kinetic energy, usually given in electron volts. The term temperature is used, since hot, thermal and cold neutrons are moderated in a medium with a certain temperature. The neutron energy distribution is then adopted to the Maxwellian distribution known for thermal motion. Qualitatively, the higher the temperature, the higher the kinetic energy is of the free neutron. Kinetic energy, speed and wavelength of the neutron are related through the De Broglie relation.
Moderated and other, non-thermal neutron energy distributions or ranges are
- Fast neutrons with kinetic energies greater than 1 eV, 0.1 MeV or approximately 1 MeV, depending on the definition.
- Slow neutrons a kinetic energy less than or equal to 0.4 eV.
- Epithermal neutrons an energy from 1 eV to 10 keV.
- Hot neutrons an energy of about 0.2 eV.
- Thermal neutrons an energy of about 0.025 eV.[29] This is the most probable energy, while the average energy is 0.038 eV.
- Cold neutrons an energy from 5 × 10−5 eV to 0.025 eV.
- Very cold neutrons an energy from 3 × 10−7 eV to 5 × 10−5 eV.
- Ultra cold neutrons an energy less than 3 × 10−7 eV.
- Continuum region neutrons an energy from 0.01 MeV to 25 MeV.
- Resonance region neutrons an energy from 1 eV to 0.01 MeV.
- Low energy region neutrons an energy less than 1 eV.
Electrons
Notation: WN5 is a component of V444 Cygni, with its Wolf-Rayet (W) spectrum dominated by NitrogenIII-V and HeliumI-II lines and WN2 to WN5 considered hotter or "early".
"The color temperature of the central part of the WN5 disk for λ < 7512 Å, where the main source of opacity is electron scattering, is Tc = 80,000-100,000 K. This high temperature represents the electron temperature slightly below the surface of the WN5 core--the level at which the star becomes optically thick in electron scattering."[30]
Muons
The colors chosen for the image on the right relate to density of the rock.
Neutrinos
Neutrino oscillation is a quantum mechanical phenomenon predicted by Bruno Pontecorvo[31] whereby a neutrino created with a specific lepton flavor (electron, muon or tau) can later be measured to have a different flavor. The probability of measuring a particular flavor for a neutrino varies periodically as it propagates. Neutrino oscillation is of theoretical and experimental interest since observation of the phenomenon implies that the neutrino has a non-zero mass.
Hard gamma rays
"Fermi's Large Area Telescope (LAT) scans the entire sky every three hours, continually deepening its portrait of the sky in gamma rays, the most energetic form of light. While the energy of visible light falls between about 2 and 3 electron volts, the LAT detects gamma rays with energies ranging from 20 million to more than 300 billion electron volts (GeV)."[32]
"At higher energies, gamma rays are rare. Above 10 GeV, even Fermi's LAT detects only one gamma ray every four months from some sources."[32]
"Any object producing gamma rays at these energies is undergoing extraordinary astrophysical processes. More than half of the 496 sources [the Fermi hard-source list] in the new census are active galaxies, where matter falling into a supermassive black hole powers jets that spray out particles at nearly the speed of light."[32]
"One example is the well-known radio galaxy NGC 1275 [above left], which is a bright, isolated source below 10 GeV. At higher energies it fades appreciably and another nearby source begins to appear. Above 100 GeV, NGC 1275 becomes undetectable by Fermi, while the new source, the radio galaxy IC 310, shines brightly."[32]
"The catalog serves as an important roadmap for ground-based facilities called Atmospheric Cherenkov Telescopes, which have amassed about 130 gamma-ray sources with energies above 100 GeV. They include the Major Atmospheric Gamma Imaging Cherenkov telescope (MAGIC) on La Palma in the Canary Islands, the Very Energetic Radiation Imaging Telescope Array System (VERITAS) in Arizona, and the High Energy Stereoscopic System (H.E.S.S.) in Namibia."[32]
Soft gamma rays
On the right is a "view of 4C 71.07 from observations by the Burst and Transient Source Experiment. This helped convince scientists that they were studying data from the quasar and not some other source in the neighborhood."[33]
"Angela [Malizia] has now discovered this quasar in soft gamma rays."[34]
"It is also known as QSO 0836+710, a quasar or quasi-stellar object that emits baffling amounts of radio energy. (The numbers actually designate the same place in the sky: 71.07 is its declination, and 0836+710 is right ascension and declination.)"[33]
"It's basically the nucleus of a galaxy that is showing extraordinary activity."[34]
"What BATSE has discovered is that it can be a soft gamma-ray source."[34]
"This makes it the faintest and most distant object to be observed in soft gamma rays. 4C 71.07 has already been observed in gamma rays by the Energetic Gamma Ray Telescope (EGRET) also aboard the Compton Gamma Ray Observatory."[33]
"In the case of 4C 71.07, it's the brightest AGN seen above 20,000 electron volts (20 keV). Its average flux (the amount of radiation reaching our telescopes) is about 13 milliCrabs, or 13/1,000ths as much as the Crab Nebula, a standard candle in astrophysics."[33]
Hard X-rays
From 0.1 nm to 0.01 nm (about 12 to 120 keV) [are] hard X-rays.
The gamma-ray source Geminga, shown at right in hard X-rays by the satellite XMM Newton, is first observed by the Second Small Astronomy Satellite (SAS-2).
Geminga may be a sort of neutron star: the decaying core of a massive star that exploded as a supernova about 300,000 years ago.[35]
This nearby explosion may be responsible for the low density of the interstellar medium in the immediate vicinity of the Solar System. This low-density area is known as the Local Bubble.[36] Possible evidence for this includes findings by the Arecibo Observatory that local micrometre-sized interstellar meteor particles appear to originate from its direction.[37]
"Geminga is a very weak neutron star and the pulsar next to us, which almost only emits extremely hard gamma-rays, but no radio waves. ... Some thousand years ago our Sun entered this [Local Bubble] several hundred light-years big area, which is nearly dust-free."[38]
Soft X-rays
From 10 to 0.1 nanometers (nm) (about 0.12 to 12 keV) they are classified as soft x-rays.
On the right is a soft X-ray image in the titanium-polyimide ("Ti_poly") filter from the Hinode X-Ray Telescope (XRT) obtained at: 2009/10/15 18:03 UTC.
"The primary filter for the sigmoid observations was the “thin-aluminum/polyimide” (or “Al/poly”) filter, imaging plasmas with temperature of roughly 2–5 MK in the active region."[39]
Super soft X-rays
Super soft X-ray source "SSXSs are in most cases only detected below 0.5 keV".[40]
There are three SSXSs with bolometric luminosity of ~1038 erg/s that are novae: GQ Mus (BB, MW), V1974 Cyg (WD, MW), and Nova LMC 1995 (WD).[41] "Apparently, as of 1999 the orbital period of Nova LMC 1995 if a binary was not known."[40]
U Sco, a recurrent nova as of 1999 unobserved by ROSAT, is a WD (74-76 eV), Lbol ~ (8-60) x 1036 erg/s, with an orbital period of 1.2306 d.[41]
"[S]uper-soft X-rays [are] between 0.12 and 2.0 keV."[42]
The image on the right contains counts from super soft X-rays.
"The first detection of Pluto in X-rays has been made using NASA's Chandra X-ray Observatory in conjunction with observations from NASA's New Horizons spacecraft."[43]
"There is a significant difference in scale between the optical and X-ray images. New Horizons made a close flyby of Pluto but Chandra is located near the Earth, so the level of detail visible in the two images is very different. The Chandra image is 180,000 miles across at the distance of Pluto, but the planet is only 1,500 miles across. Pluto is detected in the X-ray image as a point source, showing the sharpest level of detail available for Chandra or any other X-ray observatory."[43]
"Detecting X-rays from Pluto is a somewhat surprising result given that Pluto - a cold, rocky world without a magnetic field - has no natural mechanism for emitting X-rays. However, scientists knew from previous observations of comets that the interaction between the gases surrounding such planetary bodies and the solar wind - the constant streams of charged particles from the sun that speed throughout the solar system -- can create X-rays."[43]
"The immediate mystery is that Chandra's readings on the brightness of the X-rays are much higher than expected from the solar wind interacting with Pluto's atmosphere. The Chandra detection is also surprising since New Horizons discovered Pluto's atmosphere was much more stable than the rapidly escaping, "comet-like" atmosphere that many scientists expected before the spacecraft flew past in July 2015. In fact, New Horizons found that Pluto's interaction with the solar wind is much more like the interaction of the solar wind with Mars, than with a comet. While Pluto is releasing enough gas from its atmosphere to make the observed X-rays, there isn't enough solar wind flowing directly at Pluto at its great distance from the Sun to make them according to certain theoretical models."[43]
Ultra soft X-rays
Ultra-soft X-rays are also known as grenz-rays (GRs).[44]
"Ultra soft X-ray spectra [are] in the range 60-250 eV".[45]
Extreme ultraviolets
"A full-disk multiwavelength extreme ultraviolet image of the sun [was] taken by SDO on March 30, 2010. False colors trace different gas temperatures. Reds are relatively cool (about 60,000 Kelvin, or 107,540 F); blues and greens are hotter (greater than 1 million Kelvin, or 1,799,540 F)."[46]
"Some of the images from the spacecraft show never-before-seen detail of material streaming outward and away from sunspots. Others show extreme close-ups of activity on the sun’s surface. The spacecraft also has made the first high-resolution measurements of solar flares in a broad range of extreme ultraviolet wavelengths."[46]
"The Extreme Ultraviolet Variability Experiment measures fluctuations in the sun’s radiant emissions. These emissions have a direct and powerful effect on Earth’s upper atmosphere -- heating it, puffing it up, and breaking apart atoms and molecules."[46]
Far ultraviolets
"This unusual false-color image [at right] shows how the Earth glows in ultraviolet (UV) light. The Far UV Camera/Spectrograph deployed and left on the Moon by the crew of Apollo 16 captured this image. The part of the Earth facing the Sun reflects much UV light and bands of UV emission are also apparent on the side facing away from the Sun. These bands are the result of aurora caused by charged particles given off by the Sun. They spiral towards the Earth along Earth's magnetic field lines."[47]
"An artificially reproduced color enhancement [at right] of a ten-minute far-ultraviolet exposure of Earth, taken with a filter which blocks the glow caused by atomic hydrogen but which transmits the glow caused by atomic oxygen and molecular nitrogen. Note that airglow emission bands are visible on the night side of Earth, one roughly centered between the two polar auroral zones and one at an angle to this extending northward toward the sunlit side of Earth. The UV camera was operated by astronaut John W. Young on the Apollo 16 lunar landing mission."[48]
Middle ultraviolets
"Spectra of Venus (~35 Å resolution) and Jupiter (~50 Å resolution) were obtain using objective grating spectrographs in the 2300-3700 Å wavelength range. The geometric reflectivity of Jupiter, as a function of wavelength, lies in the range 0.15 to 0.25; that of Venus, 0.08 to 0.40."[49]
Near ultraviolets
"This composite image [on the right] shows Z Camelopardalis, or Z Cam, a double-star system featuring a collapsed, dead star, called a white dwarf, and a companion star, as well as a ghostly shell around the system. The massive shell provides evidence of lingering material ejected during and swept up by a powerful classical nova explosion that occurred probably a few thousand years ago."[50]
"The image combines data gathered from the far-ultraviolet and near-ultraviolet detectors on NASA's Galaxy Evolution Explorer on Jan. 25, 2004. The orbiting observatory first began imaging Z Cam in 2003."[50]
"Z Cam is the largest white object in the image, located near the center. Parts of the shell are seen as a lobe-like, wispy, yellowish feature below and to the right of Z Cam, and as two large, whitish, perpendicular lines on the left."[50]
"Z Cam was one of the first known recurrent dwarf nova, meaning it erupts in a series of small, "hiccup-like" blasts, unlike classical novae, which undergo a massive explosion."[50]
The "huge shell around Z Cam [...] it could only be explained as the remnant of a full-blown classical nova explosion. This finding provides the first evidence that some binary systems undergo both types of explosions. Previously, a link between the two types of novae had been predicted, but there was no evidence to support the theory."[50]
"The faint bluish streak in the bottom right corner of the image is ultraviolet light reflected by dust that may or may not be related to Z Cam."[50]
"The yellow objects are strong near-ultraviolet emitters; blue features have strong far-ultraviolet emission; and white objects have nearly equal amounts of near-ultraviolet and far-ultraviolet emission."[50]
Opticals
A Photometric system is a set of well-defined passbands (or filters), with a known sensitivity to incident radiation. The sensitivity usually depends on the optical system, detectors and filters used. For each photometric system a set of primary standard stars is provided.
Filter Letter | Effective Wavelength Midpoint λeff For Standard Filter[51] | Full Width Half Maximum[51] | Variant(s) | Description |
---|---|---|---|---|
Ultraviolet | ||||
U | 365nm | 66nm | u, u', u* | "U" stands for ultraviolet. |
Visible | ||||
B | 445nm | 94nm | b | "B" stands for blue. |
V | 551nm | 88nm | v, v' | "V" stands for visual. |
G | g, g' | "G" stands for green (visual). | ||
R | 658nm | 138nm | r, r', R', Rc, Re, Rj | "R" stands for red. |
Near-Infrared | ||||
I | 806nm | 149nm | i, i', Ic, Ie, Ij | "I" stands for infrared. |
Visuals
Grey or gray is an intermediate color between black and white, a neutral or achromatic color, meaning literally a color "without color." [52] It is the color of a cloud-covered sky, of ash and of lead.[53]
The first image at right shows some red pebbles among gray pebbles, which are all the same rock type.
The first image at left shows the various shades of grey.
White is the color of fresh milk and snow.[53] "of the colour of fresh milk or snow." See also Webster's New World Dictionary of American English, Third College Edition, (1988): "Having the color of pure snow or milk." See also The Random House College Dictionary of the English Language, Revised Edition,(1980). It is the color the human eye sees when it looks at light which contains all the wavelengths of the visible spectrum, at full brightness and without absorption. It does not have any hue.[53]
"de la couleur de la neige, du lait. Lumiere resultant de la combinaison de toutes les couleurs du spectre solaire."[54] (of the color of snow, of milk. Light resulting from the combination of all the colors of the solar spectrum.)
The white cliffs shown in the image at right are made of chalk, or calcium carbonate.
White light reflected off objects can be seen when no part of the light spectrum is reflected significantly more than any other and the reflecting material has a degree of diffusion. People see this when transparent fibers, particles, or droplets are in a transparent matrix of a substantially different refractive index. Examples include classic "white" substances such as sugar, foam, pure sand or snow, cotton, clouds, and milk. Crystal boundaries and imperfections can also make otherwise transparent materials white, as in the milky quartz or the microcrystalline structure of a seashell.
Cumulus clouds look white because the water droplets reflect and scatter the sunlight without absorbing other colors.
White light is generated by the sun, by stars, or by earthbound sources such as incandescent lamps, fluorescent lamps and white LEDs.
Black is the color of coal, ebony, and of outer space. It is the darkest color, the result of the absence of or complete absorption of light. It is the opposite of white and often represents darkness in contrast with light.[53][55][56]
"Opposite to white: colourless from the absence or complete absorption of light. Also, so near this as to have no distinguishable colour, very dark."[53]
Black is "[t]he darkest color".[55]
"Se dit de la couleur la plus foncée, due à l'absence ou à l'absorption totale des rayons lumineux."[56]("said of the very darkest color, due to the absence or complete absorption of all rays of light.")
Human color visions
At right are normalized absorption spectra of the three human photopsins and of human rhodopsin.
Iodopsins are the photoreceptor proteins found in the cone cells of the [human] retina that are the basis of color vision. Iodopsins are very close analogs of the visual purple rhodopsin [R] that is used in night vision. Iodopsins consist of a protein called photopsin and a bound chromophore, retinal.
In humans there are three different iodopsins (rhodopsin analogs) that form the protein-pigment complexes photopsin I, II, and III. They are called erythrolabe, chlorolabe, and cyanolabe, respectively.[57] These photopsins have absorption maxima for yellowish-green (photopsin I), green (photopsin II), and bluish-violet light (photopsin III).
Cyanolabe, or tritan, has an absorption range of 400 to 500 nm and a peak absorption wavelength of 420-440 nm.[58][59]
In humans the OPN1SW gene (GeneID: 611) encodes cyanolabe.[60][61][62]
Color | Frequency | Wavelength |
---|---|---|
violet | 668–789 THz | 380–450 nm |
blue | 631–668 THz | 450–475 nm |
cyan | 606–630 THz | 476–495 nm |
green | 526–606 THz | 495–570 nm |
yellow | 508–526 THz | 570–590 nm |
orange | 484–508 THz | 590–620 nm |
red | 400–484 THz | 620–750 nm |
The visible spectrum is the portion of the electromagnetic spectrum that is visible to (can be detected by) the human eye. Electromagnetic radiation in this range of wavelengths is called visible light or simply light. A typical human eye will respond to wavelengths from about 390 to 750 nm.[63] In terms of frequency, this corresponds to a band in the vicinity of 400–790 THz. A light-adapted eye generally has its maximum sensitivity at around 555 nm (540 THz), in the green region of the optical spectrum (see: luminosity function).
There are several cases of astronomers who claimed that following a cataract operation, they could see shorter wavelengths than other people, slightly into the ultraviolet.
Color indexes
Class | B–V | V–R | Teff (K) |
---|---|---|---|
O5V | –0.33 | –0.15 | 42,000 |
B0V | –0.30 | –0.13 | 30,000 |
A0V | –0.02 | 0.02 | 9,790 |
F0V | 0.30 | 0.30 | 7,300 |
G0V | 0.58 | 0.50 | 5,940 |
K0V | 0.81 | 0.64 | 5,150 |
M0V | 1.40 | 1.28 | 3,840 |
"[T]he [visual] color index is a simple numerical expression that determines the color of an object, which in the case of a star gives its temperature. To measure the [visual] index, one observes the magnitude of an object successively through two different filters, such as ... B and V, where ... B is sensitive to blue light, and V is sensitive to visible (green-yellow) light ... The difference in magnitudes found with these filters is called the ... B–V color index ... The smaller the color index, the more blue (or hotter) the object is. Conversely, the larger the color index, the more red (or cooler) the object is. ... For comparison, the yellowish Sun has a B–V index of 0.656 ± 0.005,[65] while the bluish Rigel has a B–V of –0.03 (its B magnitude is 0.09 and its V magnitude is 0.12, B–V = –0.03).[66]
Color indices of distant objects are usually affected by interstellar extinction —i.e. they are redder than those of closer stars. The amount of reddening is characterized by color excess, defined as the difference between the Observed color index and the Normal color index (or Intrinsic color index), the hypothetical true color index of the star, unaffected by extinction. For example, we can write it for the B-V color:
- <math>E_{B-V} = (B-V)_{\textrm{Observed}} - (B-V)_{\textrm{Intrinsic}}</math>
Abney effect
The Abney effect describes the perceived hue shift that occurs when white light is added to a monochromatic light source.[67]
The addition of white light will cause a desaturation of the monochromatic source, as perceived by the human eye. However, a less intuitive effect of the white light addition that is perceived by the human eye is the change in the apparent hue. This hue shift is physiological rather than physical in nature.
A white light source is created by the combination of red light, blue light, and green light.
The term hue discrimination is used to describe the change in wavelength that must be obtained in order for the eye to detect a shift in hue. An expression λ + Δλ defines the required wavelength adjustment that must take place.[68] A less than two nanometer change in wavelength causes most spectral colors to appear to take on a different hue. However, for blue light and red light, a much larger wavelength shift must occur in order for a person to be able to identify a difference in hue.
A white point (often referred to as reference white or target white in technical documents) is a set of tristimulus values or chromaticity coordinates that serve to define the color "white" in image capture, encoding, or reproduction.[69] Depending on the application, different definitions of white are needed to give acceptable results. For example, photographs taken indoors may be lit by incandescent lights, which are relatively orange compared to daylight. Defining "white" as daylight will give unacceptable results when attempting to color-correct a photograph taken with incandescent lighting.
Violets
Def. a “bluish-purple colour"[70] is called violet.
Def. a “colour/color that is a dark blend of red and blue; dark magenta"[71] is called purple.
Purple is a range of hues of color occurring between red and blue.[72] The Oxford English Dictionary describes it as a deep, rich shade between crimson and violet.[73]
Blues
Blue is the colour of the clear sky and the deep sea.[74]
"De la couleur du ciel sans nuages, de l'azur"[75]
Cyans
Electric blue is a color close to cyan that is a representation of the color of lightning, an electric spark, and argon signs.
The electric blue glow of electricity results from the spectral emission of the excited ionized atoms (or excited molecules) of air (mostly oxygen and nitrogen) falling back to unexcited states, which happens to produce an abundance of electric blue light. This is the reason electrical sparks in air, including lightning, appear electric blue. It is a coincidence that the color of Cherenkov radiation and light emitted by ionized air are a very similar blue despite their very different methods of production.
Aero blue is a fluorescent cyan color.
The word cerulean is probably derived from the Latin word caeruleus, "dark blue, blue or blue-green", which in turn probably derives from caelulum, diminutive of caelum, "heaven, sky".[76]
Natural gas (methane) has a cyan colored flame when burned with a mixture of air.
Greens
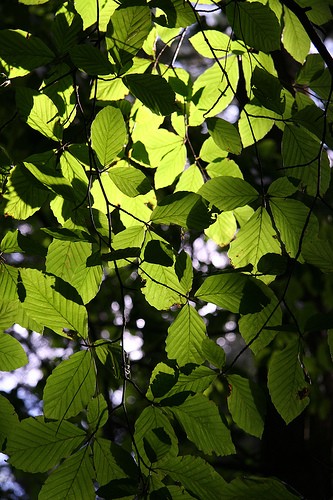
Green has a wavelength range of approximately 520–570 nm, a frequency range of ~575–525 THz, with color coordinates of (0, 255, 0) and a hexagonal triplet of #00FF00 from sRGB source of sRGB approximation to NCS S 2060-G.[78]
Def. the "colour of growing foliage, as well as other plant cells containing chlorophyll; the colour between yellow and blue in the visible spectrum; one of the primary additive colour for transmitted light; the colour obtained by subtracting red and blue from white light using cyan and yellow filters"[79] is called green.
"...in nature chiefly conspicuous as the colour of growing herbage and leaves..."[80]
"Green is the color of emeralds, jade, and growing grass.[80] In the continuum of colors of visible light it is located between yellow and blue. Green is the color most commonly associated with nature and the environmental movement, Islam, spring, hope and envy.[81]
Green is the color you see when you look at light with a wavelength of roughly 520–570 nanometers.
It is one of the three additive colors, along with red and blue, which are combined on computer screens and color televisions to make all other colors.
In the subtractive color system, used in printing, it is not a primary color, but is created out of a mixture of yellow and blue, or yellow and cyan.
On the HSV color wheel, also known as the RGB color wheel, the complement of green is magenta; that is, a purple color corresponding to an equal mixture of red and blue light. On a color wheel based on traditional color theory (RYB), the complementary color to green is considered to be red.[82]
The perception of greenness (in opposition to redness forming one of the opponent mechanisms in human color vision) is evoked by light which triggers the medium-wavelength M cone cells in the eye more than the long-wavelength L cones. Light which triggers this greenness response more than the yellowness or blueness of the other color opponent mechanism is called green. A green light source typically has a spectral power distribution dominated by energy with a wavelength of roughly 487–570 nm. More specifically, "blue green" 487–493 nm, "bluish green" 493–498 nm, "green" 498–530 nm, "yellowish green" 530–559 nm, "yellow green" 559–570 nm.[83]
Green earth is a natural pigment. It s composed of clay colored by iron oxide, magnesium, aluminum silicate, or potassium. Large deposits were found in the South of France near Nice, and in Italy around Verona, on Cyprus, and in Bohemia. The clay was crushed, washed to remove impurities, then powdered. It was sometimes called Green of Verona.[84]
Yellows
Def. "[t]he colour of gold or butter; the colour obtained by mixing green and red light, or by subtracting blue from white light"[85] is called yellow.
Def. "a bright yellow colour, resembling the metal gold"[86]
is called
gold. |
Yellow, in the form of yellow ochre pigment made from clay, was one of the first colors used in prehistoric cave art. The cave of Lascaux has an image of a horse colored with yellow estimated to be 17,300 years old.
Shades of yellow contains a more diverse set of yellow or yellow-like colors.
Oranges
Template:Shades of orange Template:Shades of brown
Def. the "colour of a ripe orange (the fruit); a color midway between red and yellow"[87] is called orange.
Reds
Template:Shades of red Template:Shades of magenta Template:Shades of pink In wavelengths, red astronomy covers 620 - 750 nm.
Far reds
Far-red light is light at the extreme red end of the visible spectrum, between red and infra-red light. Usually regarded as the region between 710 and 850 nm wavelength, it is dimly visible to some human eyes.
Infrared or red radiation from a common household radiator or electric heater is an example of thermal radiation, as is the heat emitted by an operating incandescent light bulb. Thermal radiation is generated when energy from the movement of charged particles within atoms is converted to electromagnetic radiation.
Infrared (IR) light is electromagnetic radiation with longer wavelengths than those of visible light, extending from the nominal red edge of the visible spectrum at 700 nanometres (nm) to 1 mm. This range of wavelengths corresponds to a frequency range of approximately 430 THz down to 300 GHz,[88] and includes most of the thermal radiation emitted by objects near room temperature. Infrared light is emitted or absorbed by molecules when they change their rotational-vibrational movements.
Infrareds
The wavelength of infrared light ranges from 0.75 to 300 micrometers. Infrared falls in between visible radiation, which ranges from 380 to 750 nanometers, and submillimeter waves. Infrared rays can be emitted, fluoresced, or reflected by an astronomical object.
Def. electromagnetic radiation of a wavelength longer than visible light, but shorter than microwave radiation, having a wavelength between 700 nm and 1 mm is called infrared.
Def. [e]lectromagnetic radiation having a wavelength approximately between 1 micrometre and 1 millimetre; perceived as heat is called infrared radiation.
Astronomers typically divide the infrared spectrum as follows:[89]
Designation | Abbreviation | Wavelength |
---|---|---|
Near Infrared | NIR | (0.7–1) to 5 µm |
Mid Infrared | MIR | 5 to (25–40) µm |
Far Infrared | FIR | (25–40) to (200–350) µm. |
These are the approximate ranges for photon energies of the infrared bands:
Division Name | Wavelength | Photon Energy |
Near-infrared | 0.75-1.4 µm | 0.9-1.7 eV |
Short-wavelength infrared | 1.4-3 µm | 0.4-0.9 eV |
Mid-wavelength infrared | 3-8 µm | 150-400 meV |
Long-wavelength infrared | 8–15 µm | 80-150 meV |
Far infrared | 15 - 1,000 µm | 1.2-80 meV |
Wavelength range (micrometres) |
Astronomical bands | Telescopes |
---|---|---|
0.65 to 1.0 | R and I bands | All major optical telescopes |
1.1 to 1.4 | J band | Most major optical telescopes and most dedicated infrared telescopes |
1.5 to 1.8 | H band | Most major optical telescopes and most dedicated infrared telescopes |
2.0 to 2.4 | K band | Most major optical telescopes and most dedicated infrared telescopes |
3.0 to 4.0 | L band | Most dedicated infrared telescopes and some optical telescopes |
4.6 to 5.0 | M band | Most dedicated infrared telescopes and some optical telescopes |
7.5 to 14.5 | N band | Most dedicated infrared telescopes and some optical telescopes |
17 to 25 | Q band | Some dedicated infrared telescopes and some optical telescopes |
28 to 40 | Z band | Some dedicated infrared telescopes and some optical telescopes |
330 to 370 | Some dedicated infrared telescopes and some optical telescopes | |
450 | submillimeter | Submillimeter telescopes |
The infrared band may be divided up based on the response of various detectors:[90]
- Near infrared: from 0.7 to 1.0 µm (from the approximate end of the response of the human eye to that of silicon).
- Short-wave infrared: 1.0 to 3 µm (from the cut off of silicon to that of the MWIR atmospheric window. InGaAs covers to about 1.8 µm; the less sensitive lead salts cover this region.
- Mid-wave infrared: 3 to 5 µm (defined by the atmospheric window and covered by indium antimonide [InSb] and HgCdTe and partially by lead selenide [PbSe]).
- Long-wave infrared: 8 to 12, or 7 to 14 µm: the atmospheric window (Covered by HgCdTe and microbolometers).
- Very-long wave infrared (VLWIR): 12 to about 30 µm, covered by doped silicon.
A commonly used sub-division scheme is:[91]
Division Name | Abbreviation | Wavelength | Characteristics |
Near-infrared | NIR, IR-A DIN | 0.75-1.4 µm | Defined by the water absorption, and commonly used in fiber optic telecommunication because of low attenuation losses in the SiO2 glass (silica) medium. Image intensifiers are sensitive to this area of the spectrum. Examples include night vision devices such as night vision goggles. |
Short-wavelength infrared | SWIR, IR-B DIN | 1.4-3 µm | Water absorption increases significantly at 1,450 nm. The 1,530 to 1,560 nm range is the dominant spectral region for long-distance telecommunications. |
Mid-wavelength infrared | MWIR, IR-C DIN. Also called intermediate infrared (IIR) | 3-8 µm | In guided missile technology the 3-5 µm portion of this band is the atmospheric window in which the homing heads of passive IR 'heat seeking' missiles are designed to work, homing on to the Infrared signature of the target aircraft, typically the jet engine exhaust plume |
Long-wavelength infrared | LWIR, IR-C DIN | 8–15 µm | This is the "thermal imaging" region, in which sensors can obtain a completely passive picture of the outside world based on thermal emissions only and requiring no external light or thermal source such as the sun, moon or infrared illuminator. Forward-looking infrared (FLIR) systems use this area of the spectrum. This region is also called the "thermal infrared." |
Far infrared | FIR | 15 - 1,000 µm | (see also far-infrared laser). |
NIR and SWIR is sometimes called "reflected infrared" while MWIR and LWIR is sometimes referred to as "thermal infrared." Due to the nature of the blackbody radiation curves, typical 'hot' objects, such as exhaust pipes, often appear brighter in the MW compared to the same object viewed in the LW.
Submillimeters
“[T]erahertz radiation refers to electromagnetic waves propagating at frequencies in the terahertz range. It is synonymously termed submillimeter radiation, terahertz waves, terahertz light, T-rays, T-waves, T-light, T-lux, THz. The term typically applies to electromagnetic radiation with frequencies between high-frequency edge of the microwave band, 300 gigahertz (3 x 1011 Hz),"[92] and the long-wavelength edge of far-infrared light, 3000 GHz (3 x 1012 Hz or 3 THz). In wavelengths, this range corresponds to 0.1 mm (or 100 µm) infrared to 1.0 mm microwave.
Terahertz radiation is emitted as part of the black body radiation from anything with temperatures greater than about 10 kelvin. While this thermal emission is very weak, observations at these frequencies are important for characterizing the cold 10-20K dust in the interstellar medium in the Milky Way galaxy, and in distant starburst galaxies. Telescopes operating in this band include the James Clerk Maxwell Telescope, the Caltech Submillimeter Observatory and the Submillimeter Array at the Mauna Kea Observatory in Hawaii, the BLAST balloon borne telescope, the Herschel Space Observatory, and the Heinrich Hertz Submillimeter Telescope at the Mount Graham International Observatory in Arizona. The Atacama Large Millimeter Array, under construction, will operate in the submillimeter range. The opacity of the Earth's atmosphere to submillimeter radiation restricts these observatories to very high altitude sites, or to space.
Microwaves
The figure at the right "shows the three-year [Wilkinson Microwave Anisotropy Probe] WMAP spectrum compared to a set of recent balloon and ground-based measurements that were selected to most complement the WMAP data in terms of frequency coverage and l range. The non-WMAP data points are plotted with errors that include both measurement uncertainty and cosmic variance, while the WMAP data in this l range are largely noise dominated, so the effective error is comparable. When the WMAP data are combined with these higher resolution CMB measurements, the existence of a third acoustic peak is well established, as is the onset of Silk damping beyond the 3rd peak."[93]
Microwaves, a subset of radio waves, have wavelengths ranging from as long as one meter to as short as one millimeter, or equivalently, with frequencies between 300 MHz (0.3 GHz) and 300 GHz.[94] This broad definition includes both [ultra high frequency] UHF and [extremely high frequency] EHF (millimeter waves), and various sources use different boundaries.[95] In all cases, microwave includes the entire [super high frequency] SHF band (3 to 30 GHz, or 10 to 1 cm) at minimum, with [radio frequency] RF engineering often putting the lower boundary at 1 GHz (30 cm), and the upper around 100 GHz (3 mm).
Letter Designation | Frequency range | Wavelength range | Typical uses |
---|---|---|---|
L band | 1 to 2 GHz | 15 cm to 30 cm | military telemetry, GPS, mobile phones (GSM), amateur radio |
S band | 2 to 4 GHz | 7.5 cm to 15 cm | weather radar, surface ship radar, and some communications satellites (microwave ovens, microwave devices/communications, radio astronomy, mobile phones, wireless LAN, Bluetooth, ZigBee, GPS, amateur radio) |
C band | 4 to 8 GHz | 3.75 cm to 7.5 cm | long-distance radio telecommunications |
X band | 8 to 12 GHz | 25 mm to 37.5 mm | satellite communications, radar, terrestrial broadband, space communications, amateur radio |
Ku band or P band | 12 to 18 GHz | 16.7 mm to 25 mm | satellite communications |
K band | 18 to 26.5 GHz | 11.3 mm to 16.7 mm | radar, satellite communications, astronomical observations, automotive radar |
Ka band | 26.5 to 40 GHz | 5.0 mm to 11.3 mm | satellite communications |
Q band | 33 to 50 GHz | 6.0 mm to 9.0 mm | satellite communications, terrestrial microwave communications, radio astronomy, automotive radar |
U band | 40 to 60 GHz | 5.0 mm to 7.5 mm | |
V band | 50 to 75 GHz | 4.0 mm to 6.0 mm | millimeter wave radar research and other kinds of scientific research |
W band | 75 to 110 GHz | 2.7 mm to 4.0 mm | satellite communications, millimeter-wave radar research, military radar targeting and tracking applications, and some non-military applications, automotive radar |
F band | 90 to 140 GHz | 2.1 mm to 3.3 mm | SHF transmissions: Radio astronomy, microwave devices/communications, wireless LAN, most modern radars, communications satellites, satellite television broadcasting, DBS, amateur radio |
D band | 110 to 170 GHz | 1.8 mm to 2.7 mm | EHF transmissions: Radio astronomy, high-frequency microwave radio relay, microwave remote sensing, amateur radio, directed-energy weapon, millimeter wave scanner |
Radars
Radio waves are a type of electromagnetic radiation with wavelengths in the electromagnetic spectrum longer than infrared light. Radio waves have frequencies from 300 Gigahertz GHz to as low as 3 Kilohertz kHz, and corresponding to wavelengths from 1 millimeter to 100 kilometers.
Band name | Frequency range | Wavelength range | Notes |
---|---|---|---|
HF | 3–30 MHz | 10–100 m | Coastal radar systems, over-the-horizon radar (OTH) radars; 'high frequency' |
VHF | 30–300 MHz | 1–10 m | Very long range, ground penetrating; 'very high frequency' |
P | < 300 MHz | > 1 m | 'P' for 'previous', applied retrospectively to early radar systems; essentially HF + VHF |
UHF | 300–1000 MHz | 0.3–1 m | Very long range (e.g. ballistic missile early warning), ground penetrating, foliage penetrating; 'ultra high frequency' |
L | 1–2 GHz | 15–30 cm | Long range air traffic control and surveillance; 'L' for 'long' |
S | 2–4 GHz | 7.5–15 cm | Moderate range surveillance, Terminal air traffic control, long-range weather, marine radar; 'S' for 'short' |
C | 4–8 GHz | 3.75–7.5 cm | Satellite transponders; a compromise (hence 'C') between X and S bands; weather; long range tracking |
X | 8–12 GHz | 2.5–3.75 cm | Missile guidance, marine radar, weather, medium-resolution mapping and ground surveillance; in the USA the narrow range 10.525 GHz ±25 MHz is used for airport radar; short range tracking. Named X band because the frequency was a secret during WW2. |
Ku | 12–18 GHz | 1.67–2.5 cm | High-resolution, also used for satellite transponders, frequency under K band (hence 'u') |
K | 18–24 GHz | 1.11–1.67 cm | From German kurz, meaning 'short'; limited use due to absorption by water vapour, so Ku and Ka were used instead for surveillance. K-band is used for detecting clouds by meteorologists, and by police for detecting speeding motorists. K-band radar guns operate at 24.150 ± 0.100 GHz. |
Ka | 24–40 GHz | 0.75–1.11 cm | Mapping, short range, airport surveillance; frequency just above K band (hence 'a') Photo radar, used to trigger cameras which take pictures of license plates of cars running red lights, operates at 34.300 ± 0.100 GHz. |
mm | 40–300 GHz | 1.0–7.5 mm | Millimetre band, subdivided as below. The frequency ranges depend on waveguide size. Multiple letters are assigned to these bands by different groups. These are from Baytron, a now defunct company that made test equipment. |
V | 40–75 GHz | 4.0–7.5 mm | Very strongly absorbed by atmospheric oxygen, which resonates at 60 GHz. |
W | 75–110 GHz | 2.7–4.0 mm | Used as a visual sensor for experimental autonomous vehicles, high-resolution meteorological observation, and imaging. |
Auroras
"Electron temperatures are generally derived from the ratio of auroral to nebular lines in [O III] or [N II]."[97] "[B]ecause of the proximity of strong night-sky lines at λ4358 and λλ5770, 5791, the auroral lines of [O III] λ4363 and [N II] λ5755 are often contaminated."[97]
Coronal clouds
"The temperature of yellow coronal regions is ... about 2.5 [x] 106 [K]. ... although some ions Ca XV will exist at lower, as well as higher temperatures."[98]
"The AS prominences [AS in Menzel-Evans' classification [4];] move with velocities exceeding by far the velocities of other types of prominences [7], [8]. As short-living phenomena, they are condensed quickly and the temperature of the coronal gases should rise in the early stages of their condensation. Indeed, the AS prominences use to be allied with yellow line emission (λ 5694)."[98]
"The yellow line is namely due to the ion Ca XV, according to Edlen's and Waldmeier's identification. ... the line λ 5694 is emitted by 3P1 - 3P0 transition of Ca XV."[98]
"The solar corona is not in thermodynamical equilibrium. In particular, the photo-recombination is compensated with electron impact ionization, while the reverse processes viz. the photoionization and recombination by impact with two electrons are there negligible."[98]
Asteroids
"[B]roadband optical photometry of Centaurs and Kuiper Belt objects [KBOs] from the Keck 10 m, the University of Hawaii 2.2 m, and the Cerro Tololo InterAmerican (CTIO) 1.5 m telescopes [shows] a wide dispersion in the optical colors of the objects, indicating nonuniform surface properties. The color dispersion [may] be understood in the context of the expected steady reddening due to bombardment by the ubiquitous flux of cosmic rays."[99]
Kuiper belts
"These authors proposed that the whole-disk surface colors of KBOs could be the result of the competition between the effects of irradiation of surface organics by cosmic-rays and the global resurfacing due to impacts. [...] When these high-energy protons collide with an icy target, they penetrate very [deep] under the surface."[100]
Hypotheses
- The orthogonality between electric fields and magnetic fields came before 3D space.
- Orthogonality between electric fields and magnetic fields creates the illusion of three-dimensional space.
- Interference interactions create attractions and repulsions between fields which in turn produces radiance, motion, and allows time to be calibrated.
- Interactions create segmentation yielding an apparent particle/wave duality.
Acknowledgements
The content on this page was first contributed by: Henry A. Hoff.
Initial content for this page in some instances came from Wikiversity.
See also
References
- ↑ Principles Of Instrumental Analysis F.James Holler, Douglas A. Skoog & Stanley R. Crouch 2006
- ↑ Tom Harris. How Fluorescent Lamps Work. Discovery Communications. Retrieved 27 June 2010.
- ↑ Stokes, G. G. (1852). "On the Change of Refrangibility of Light". Philosophical Transactions of the Royal Society of London. 142: 463–562. doi:10.1098/rstl.1852.0022.
- ↑ K. Przibram (1935). "Fluorescence of Fluorite and the Bivalent Europium Ion". Nature. 135 (3403): 100. Bibcode:1935Natur.135..100P. doi:10.1038/135100a0.
- ↑ D.W. Thompson; et al. (1998). "Determination of optical anisotropy in calcite from ultraviolet to mid-infrared by generalized ellipsometry". Thin Solid Films. 313–4 (1–2): 341–6. Bibcode:1998TSF...313..341T. doi:10.1016/S0040-6090(97)00843-2.
- ↑ 6.0 6.1 6.2 D. K. Yeomans (1998). Great Comets in History. Jet Propulsion Laboratory. Retrieved 15 March 2007.
- ↑ D. A. Mendis (1988). "A Postencounter view of comets". Annual Review of Astronomy and Astrophysics. 26 (1): 11–49. Bibcode:1988ARA&A..26...11M. doi:10.1146/annurev.aa.26.090188.000303.
- ↑ Ian Ridpath (1985). Through the comet’s tail. Revised extracts from A Comet Called Halley by Ian Ridpath, published by Cambridge University Press in 1985. Retrieved 2011-06-19.
- ↑ Brian Nunnally (May 16, 2011). This Week in Science History: Halley’s Comet. pfizer: ThinkScience Now. Retrieved 2011-06-19.
- ↑ Yerkes Observatory Finds Cyanogen in Spectrum of Halley's Comet, n: The New York Times. 8 February 1910. Retrieved 15 November 2009.
- ↑ 11.0 11.1 "Ten Notable Apocalypses That (Obviously) Didn't Happen". Smithsonian magazine 2009. Retrieved 14 November 2009.
- ↑ Interesting Facts About Comets. Universe Today. 2009. Retrieved 15 January 2009.
- ↑ Heber D. Curtis (June 1910). "Photographs of Halley's Comet made at the Lick Observatory". Publications of the Astronomical Society of the Pacific. 22 (132): 117–30. Bibcode:1910PASP...22..117C.
|access-date=
requires|url=
(help) - ↑ Robert Roy Britt (2001-11-29). Comet Borrelly Puzzle: Darkest Object in the Solar System. Space.com. Retrieved 2012-09-01.
- ↑ Tony Hoffman. Comet West: The Great Comet of 1976. Earthlink. Retrieved 2013-05-02.
- ↑ Abraham Loeb, Mark J. Reid, Andreas Brunthaler, and Heino Falcke (November 2005). "Constraints on the Proper Motion of the Andromeda Galaxy Based on the Survival of Its Satellite M33". The Astrophysical Journal. 633 (2): 894–8. Bibcode:2005ApJ...633..894L. doi:10.1086/491644. Retrieved 2011-11-14.
- ↑ The Grand Collision, from the series: The Sky At Night, airdate: November 5, 2007
- ↑ Cox, T. J.; Loeb, A. (2008). "The collision between the Milky Way and Andromeda". Monthly Notices of the Royal Astronomical Society. 386 (1): 461–474. Bibcode:2008MNRAS.tmp..333C. doi:10.1111/j.1365-2966.2008.13048.x.
- ↑ Cain, F. (2007). When Our Galaxy Smashes Into Andromeda, What Happens to the Sun?. Universe Today. Retrieved 2007-05-16.
- ↑ 20.0 20.1 20.2 20.3 Mark Fischetti (February 5, 2019). Warning Scale Unveiled for Dangerous Rivers in the Sky. Scientific American. Retrieved 8 February 2019.
- ↑ 21.0 21.1 Martin Ralph (February 5, 2019). Warning Scale Unveiled for Dangerous Rivers in the Sky. Scientific American. Retrieved 8 February 2019.
- ↑ 22.0 22.1 S. Swordy (2001). "The energy spectra and anisotropies of cosmic rays". Space Science Reviews. 99: 85–94.
- ↑ M. Merker, E. S. Light, R. B. Mendell and S. A. Korff (1970). A. Somogyi, ed. The flux of fast neutrons in the atmosphere. 1. The effect of solar modulation of galactic cosmic rays, In: Solar Cosmic Rays, Modulation of Galactic Radiation, Magnetospheric and Atmospheric Effects. 2. Budapest: International Conference on Cosmic Rays. p. 739. Bibcode:1970ICRC....2..739M. Retrieved 2017-08-15.
- ↑ Jörg R Hörandel, N N Kalmykov and A V Timokhin (2006). "The end of the galactic cosmic-ray energy spectrum-a phenomenological view". Journal of Physics: Conference Series. 47 (1): 132–41. doi:10.1088/1742-6596/47/1/017. Retrieved 2011-12-31. Unknown parameter
|month=
ignored (help); Unknown parameter|pdf=
ignored (help) - ↑ 25.0 25.1 25.2 25.3 E. W. Cliver, S. W. Kahler, M. A. Shea, and D. F. Smart (1982). "Injection onsets of ~2 GeV protons, ~1 MeV electrons, and ~100 keV electrons in solar cosmic ray flares". The Astrophysical Journal. 260 (9): 362–70. Bibcode:1982ApJ...260..362C. Unknown parameter
|month=
ignored (help);|access-date=
requires|url=
(help) - ↑ D.E. Alexandreas, R.C. Allen, D. Berley, S.D. Biller, R.L. Burman, D.R. Cady, C.Y. Chang, B.L. Dingus, G.M. Dion, R.W. Ellsworth, M.K. Gilra, J.A. Goodman, S. Gupta, T.J. Haines, C.M. Hoffman, D.A. Krakauer, P. Kwok, J. lloyd-Evans, D.E. Nagle, M.E. Potter, V.D. Sandberg, M.J. Stark, R.L. Talaga, P.R. Vishwanath, G.B. Yodh, W. Zhang (1991). "Observation of shadowing of ultrahigh-energy cosmic rays by the moon and the sun". Physical Review, D (Particles Fields). 43 (5): 1735–8. Retrieved 2012-08-22. Unknown parameter
|month=
ignored (help) - ↑ E. Kirsch, U.A. Mall, B. Wilken, G. Gloeckler, A.B. Galvin, and K. Cierpka (August 17, 1999). D. Kieda, M. Salamon, and B. Dingus, ed. Detection of Pickup- and Sputter Ions by Experiment SMS on the WIND-S/C After a Mercury Conjunction, In: Proceedings of the 26th International Cosmic Ray Conference. Salt Lake City, Utah, USA: International Union of Pure and Applied Physics (IUPAP). pp. 212–5. Bibcode:1999ICRC....6..212K.
|access-date=
requires|url=
(help) - ↑ 28.0 28.1 28.2 Eric R. Christian (7 April 2011). Anomalous Cosmic Rays. Greenbelt, Maryland USA: NASA Goddard Space Flight Center. Retrieved 2017-08-05.
- ↑ Atoms, Radiation, and Radiation Protection, J.E. Turner, Wiley-VCH, 2007, p. 214.
- ↑ A. M. Cherepashchuk, K. F. Khaliullin, & J. A. Eaton (June 15, 1984). "Ultraviolet photometry from the Orbiting Astronomical Observatory. XXXIX - The structure of the eclipsing Wolf-Rayet binary V444 Cygni as derived from light curves between 2460 A and 3. 5 microns". The Astrophysical Journal. 281 (06): 774–88. Bibcode:1984ApJ...281..774C. doi:10.1086/162156. Retrieved 2014-01-23.
- ↑ B. Pontecorvo (1957). "Mesonium and anti-mesonium". Zh. Eksp. Teor. Fiz. 33: 549–551. reproduced and translated in Sov. Phys. JETP. 6: 429. 1957. Missing or empty
|title=
(help) and B. Pontecorvo (1967). "Neutrino Experiments and the Problem of Conservation of Leptonic Charge". Zh. Eksp. Teor. Fiz. 53: 1717. reproduced and translated in Sov. Phys. JETP. 26: 984. 1968. Bibcode:1968JETP...26..984P. Missing or empty|title=
(help) - ↑ 32.0 32.1 32.2 32.3 32.4 Trent J. Perrotto (10 January 2012). NASA's Fermi Space Telescope Explores New Energy Extremes. Washington, DC USA: NASA. Retrieved 3 November 2016.
- ↑ 33.0 33.1 33.2 33.3 John M. Horack (18 November 1999). BATSE finds most distant quasar yet seen in soft gamma rays. Washington, DC USA: NASA. Retrieved 3 November 2016.
- ↑ 34.0 34.1 34.2 Mike McCollough (18 November 1999). BATSE finds most distant quasar yet seen in soft gamma rays. Washington, DC USA: NASA. Retrieved 3 November 2016.
- ↑ Geminga, Internet Encyclopedia of Science
- ↑ Template:Cite doi
- ↑ The Sun's Exotic Neighborhood. Centauri Dreams. 2008-02-28.
- ↑ Juergen Kummer (June 27, 2006). Geminga. Muehlenstr. 6 87474 Buchenberg Germany: Internetservice Kummer + Oster GbR. Retrieved 2013-05-08.
- ↑ D. E. McKenzie and R. C. Canfield (2008). "Hinode XRT observations of a long-lasting coronal sigmoid" (PDF). Astronomy & Astrophysics. 481: L65–L68. doi:10.1051/0004-6361:20079035. Retrieved 2016-11-03.
- ↑ 40.0 40.1 Marshallsumter (March 8, 2013). Super soft X-ray source. San Francisco, California: Wikimedia Foundation, Inc. Retrieved 2013-05-18.
- ↑ 41.0 41.1 Greiner J (2000). "Catalog of supersoft X-ray sources". New Astron. 5 (3): 137–41. arXiv:astro-ph/0005238. Bibcode:2000NewA....5..137G. doi:10.1016/S1384-1076(00)00018-X.
- ↑ Nicholas Hunt-Walker (June 2009). What Emits Astrophysical X-rays?. Madison, WI 53706: Univ. of Wisconsin - Madison. Retrieved 2016-11-03.
- ↑ 43.0 43.1 43.2 43.3 Sue Lavoie (June 2015). PIA21061: X-Rays from Pluto. Pasadena, California USA: NASA/JPL. Retrieved 2016-11-22.
- ↑ Mari-Anne Hedblad and Lotus Mallbris (2012). "Grenz ray treatment of lentigo maligna and early lentigo maligna melanoma". Journal of the American Academy of Dermatology. 67 (1): 60–8. doi:10.1016/j.jaad.2011.06.029. Retrieved 2016-09-22. Unknown parameter
|month=
ignored (help) - ↑ J. A. M. Bleeker, J. Davelaar, A. J. M. Deerenberg, H. Huizenga, A. C. Brinkman, J. Heise, Y. Tanaka, S. Hayakawa and K. Yamashita (1978). "Observation of the Ultra Soft X-ray Spectrum of HZ 43". Astronomy and Astrophysics. 69 (1): 145–148. Bibcode:1978A&A....69..145B. Retrieved 2016-11-03. Unknown parameter
|month=
ignored (help) - ↑ 46.0 46.1 46.2 Holly Zell (30 March 2010). First-light. Washington, DC USA: NASA. Retrieved 2016-11-03.
- ↑ Ruth Netting (March 22, 2011). ULTRAVIOLET LIGHT FROM OUR SUN. Washington, DC USA: NASA. Retrieved 2013-05-29.
- ↑ Amiko Kauderer (21 April 1972). Apollo Imagery. Washington, DC USA: NASA. Retrieved 2016-11-03.
- ↑ D. C. Evans, A. Boggess, III, and R. Scolnik (1965). "The Reflectivity of Venus and Jupiter in the Middle Ultraviolet". Astronomical Journal. 70: 321. Bibcode:1965AJ.....70..321E. Retrieved 2016-11-04.
- ↑ 50.0 50.1 50.2 50.3 50.4 50.5 50.6 M. Seibert, T. Pyle and R. Hurt (7 March 2007). Scene of Multiple Explosions. Pasadena, California USA: NASA/Jet Propulsion Laboratory. Retrieved 4 November 2016.
- ↑ 51.0 51.1 James Binney; Merrifield M. Galactic Astronomy, Princeton University Press, 1998, ch. 2.3.2, pp. 53
- ↑ Webster's New World Dictionary of the American Language, Third College Edition.
- ↑ 53.0 53.1 53.2 53.3 53.4 Shorter Oxford English Dictionary, 5th edition, 2002.
- ↑ Petit Larousse (2005). Missing or empty
|title=
(help);|access-date=
requires|url=
(help) - ↑ 55.0 55.1 See also Webster's New World Dictionary of the American Language (1964)
- ↑ 56.0 56.1 Le Petit Larousse Illustré (1997)
- ↑ W. A. H. Rushton (1 June 1966). "Densitometry of pigments in rods and cones of normal and color defective subjects" (PDF). Investigative Ophthalmology. 5 (3): 233–41. PMID 5296487. Retrieved 2006-11-14.
- ↑ Günther Wyszecki and W.S. Stiles (1982). Color Science: Concepts and Methods, Quantitative Data and Formulae (2nd ed.). New York: Wiley Series in Pure and Applied Optics. ISBN 0-471-02106-7.
- ↑ R. W. G. Hunt (2004). The Reproduction of Colour (6th ed.). Chichester UK: Wiley–IS&T Series in Imaging Science and Technology. pp. 11–12. ISBN 0-470-02425-9.
- ↑ Nathans J, Thomas D, Hogness DS (1986). "Molecular genetics of human color vision: the genes encoding blue, green, and red pigments". Science. 232 (4747): 193–202. doi:10.1126/science.2937147. PMID 2937147. Unknown parameter
|month=
ignored (help) - ↑ Fitzgibbon J, Appukuttan B, Gayther S, Wells D, Delhanty J, Hunt DM (1994). "Localisation of the human blue cone pigment gene to chromosome band 7q31.3-32". Human Genetics. 93 (1): 79–80. PMID 8270261. Unknown parameter
|month=
ignored (help) - ↑ Entrez Gene: OPN1SW opsin 1 (cone pigments), short-wave-sensitive (color blindness, tritan).
- ↑ Cecie Starr (2005). Biology: Concepts and Applications. Thomson Brooks/Cole. ISBN 053446226X.
- ↑ Martin V. Zombeck (1990). Calibration of MK spectral types, In: Handbook of Space Astronomy and Astrophysics (2nd ed.). Cambridge University Press. p. 105. ISBN 0-521-34787-4.
- ↑ David F. Gray (1992). "The Inferred Color Index of the Sun". Publications of the Astronomical Society of the Pacific. 104 (681): 1035–8. Bibcode:1992PASP..104.1035G. Unknown parameter
|month=
ignored (help) - ↑ Rigel.
- ↑ Pridmore, R. “Effect of purity on hue (Abney effect) in various conditions.” Color Research and Application. 32.1 (2007): 25–39.
- ↑ The Perception of Color
- ↑ Glenn Kennel (2006). Color and Mastering for Digital Cinema. Focal Press. ISBN 0-240-80874-6.
- ↑ violet. San Francisco, California: Wikimedia Foundation, Inc. February 8, 2013. Retrieved 2013-03-20.
- ↑ purple. San Francisco, California: Wikimedia Foundation, Inc. February 13, 2013. Retrieved 2013-03-20.
- ↑ Mish, Frederic C., Editor in Chief Webster's Ninth New Collegiate Dictionary Springfield, Massachusetts, U.S.A.:1984--Merriam-Webster Page 957
- ↑ Shorter Oxford English Dictionary, 5th Edition, 2003.
- ↑ See Webster's New World Dictionary of the American Language, College Edition, 1962.
- ↑ Le Petit Larousse illustré 1997. Librairie Larousse. 1996. p. 1784. ISBN 2033011976. Retrieved 2013-05-28.
- ↑ Cerulean, Online Etymology Dictionary
- ↑ Malachite. WebExhibits. 2001. Retrieved 2007-12-08.
- ↑ The sRGB values are taken by converting the NCS color 2060-G using the "NCS Navigator" tool at the NCS website.
- ↑ green. San Francisco, California: Wikimedia Foundation, Inc. July 21, 2012. Retrieved 2012-07-22.
- ↑ 80.0 80.1 (Oxford English Dictionary, 2nd Edition, Clarendon Press, Oxford, 1989.) See also first definition in Webster's New World Dictionary of the American Language, The World Publishing Company, New York, 1962.
- ↑ Eva Heller, Psychologie de la couleur- effets et symboliques. pg. 87-104
- ↑ Glossary Term: Color wheel. Sanford Corp. 2005. Archived from the original on November 2, 2007. Retrieved 2007-11-22.
- ↑ Kenneth L. Kelly (1943). "Color Designations for Lights". Journal of the Optical Society of America 33(11). 627–632.
- ↑ Anne Varichon (2000), Couleurs- pigments et teintures dans les mains des peoples. Pg. 210-211.
- ↑ yellow. San Francisco, California: Wikimedia Foundation, Inc. September 1, 2013. Retrieved 2013-09-02.
- ↑ gold. San Francisco, California: Wikimedia Foundation, Inc. August 28, 2013. Retrieved 2013-09-02.
- ↑ orange. San Francisco, California: Wikimedia Foundation, Inc. February 20, 2014. Retrieved 2014-03-01.
- ↑ S. C. Liew. Electromagnetic Waves. Centre for Remote Imaging, Sensing and Processing. Retrieved 2006-10-27.
- ↑ IPAC Staff. Near, Mid and Far-Infrared. NASA ipac. Retrieved 4 April 2007.
- ↑ Miller, Principles of Infrared Technology (Van Nostrand Reinhold, 1992), and Miller and Friedman, Photonic Rules of Thumb, 2004. isbn=9780442012106
- ↑ James Byrnes (2009). Unexploded Ordnance Detection and Mitigation. Springer. pp. 21–22. ISBN 9781402092527.
- ↑ Hankwang (October 31, 2008). Terahertz radiation. p. 1. Retrieved 2012-08-05.
- ↑ G. Hinshaw, M. R. Nolta, C. L. Bennett, R. Bean, O. Doré, M. R. Greason, M. Halpern, R. S. Hill, N. Jarosik, A. Kogut, E. Komatsu, M. Limon, N. Odegard, S. S. Meyer, L. Page, H. V. Peiris, D. N. Spergel, G. S. Tucker, L. Verde, J. L. Weiland, E. Wollack, and E. L. Wright (2007). "Three-Year Wilkinson Microwave Anisotropy Probe (WMAP1) Observations: Temperature Analysis" (PDF). The Astrophysical Journal (Supplement Series). 170 (2): 288–334. arXiv:astro-ph/0603451. Bibcode:2007ApJS..170..288H. doi:10.1086/513698. Retrieved 2014-10-19. Unknown parameter
|month=
ignored (help) - ↑ Pozar, David M. (1993). Microwave Engineering Addison-Wesley Publishing Company. ISBN 0-201-50418-9.
- ↑ http://www.google.com/search?hl=en&defl=en&q=define:microwave&ei=e6CMSsWUI5OHmQee2si1DQ&sa=X&oi=glossary_definition&ct=title
- ↑ S. Wolpert (July 24, 2008). Scientists solve 30-year-old aurora borealis mystery. University of California. Retrieved 2008-10-11.
- ↑ 97.0 97.1 S. A. Hawley (September 1, 1978). "The chemical composition of galactic and extragalactic H II regions". The Astrophysical Journal. 224 (9): 417–36. Bibcode:1978ApJ...224..417H. doi:10.1086/156389.
|access-date=
requires|url=
(help) - ↑ 98.0 98.1 98.2 98.3 J. Kleczek (1957). "Temperature of Yellow Coronal Regions". Bulletin of the Astronomical Institutes of Czechoslovakia. 8: 68–70. Bibcode:1957BAICz...8...68K. Retrieved 2013-09-26.
- ↑ Jane Luu and David Jewitt (November 1996). "Color Diversity among the Centaurs and Kuiper Belt Objects". The Astronomical Journal. 112 (5): 2310–8. Bibcode:1996AJ....112.2310L. Retrieved 2013-11-05.
- ↑ R Gil-Hutton (January 2002). "Color diversity among Kuiper belt objects: The collisional resurfacing model revisited". Planetary and Space Science. 50 (1): 57–62. Retrieved 2014-01-23.
External links
Editor-In-Chief: Henry A Hoff
- CS1 maint: Explicit use of et al.
- CS1: Julian–Gregorian uncertainty
- Pages using citations with accessdate and no URL
- CS1 maint: Multiple names: authors list
- Pages with citations using unsupported parameters
- Pages with citations lacking titles
- Articles containing French-language text
- Pages with broken file links
- Astrophysics/Lectures
- Radiation/Lectures
- Radiation astronomy/Lectures
- Resources last modified in October 2019