Mineral radiation astronomy
Editor-In-Chief: Henry A. Hoff
Uraninite is a radioactive, uranium-rich mineral and ore with a chemical composition that is largely [uranium dioxide] UO2, but also contains [uranium trioxide] UO3 and oxides of lead, thorium, and rare earth elements. It is most commonly known as pitchblende (from pitch, because of its black color. All uraninite minerals contain a small amount of radium as a radioactive decay product of uranium. Uraninite also always contains small amounts of the lead isotopes 206Pb and 207Pb, the end products of the decay series of the uranium isotopes 238U and 235U respectively. The extremely rare element technetium can be found in uraninite in very small quantities (about 0.2 ng/kg), produced by the spontaneous fission of uranium-238.
The image at left shows well-formed crystals of uraninite. The image at right shows botryoidal uraninite. Because of the uranium decay products, both sources are gamma-ray emitters.
Minerals
"A mineral is a naturally occurring homogeneous solid, inorganically formed, with a definite chemical composition and an ordered atomic arrangement. Ice is naturally occurring, given a temperature below 0 degrees Celsius (32 degrees Fahrenheit). It is homogenous (of one material), formed inorganically, and has an ordered atomic structure. Ice has a definite chemical composition (H20), with hydrogen and oxygen atoms bonding in a specific manner."[1]
Theoretical geochemistry
Def. the "branch of chemistry that deals with the chemical composition of the Earth and other planets, and with the chemical processes that occur in the formation of rocks and minerals etc"[2] is called geochemistry.
Magnetism
Def. a mineral that is weakly attracted by the poles of a magnet but does not retain any permanent magnetism is called a paramagnet, or a paramagnetic mineral.
Def. a mineral that is weakly attracted by the poles of a magnet tending to become magnetized in a direction at 180° to the applied magnetic field but does not retain any permanent magnetism is called a diamagnet, or a diamagnetic mineral.
Def. a mineral that is exhibits the poles of a magnet even in zero applied magnetic field is called a ferromagnet, or a ferromagnetic mineral.
Def. a mineral that is exhibits the poles of a magnet even in zero applied magnetic field but with opposite directions is called a antiferromagnet, or a antiferromagnetic mineral.
Def. a mineral that is exhibits the poles of a magnet even in zero applied magnetic field with opposite directions but a net magnetic moment is called a ferrimagnet, or a ferrimagnetic mineral.
Flame emission spectroscopy
In flame emission spectroscopy, as shown in the image on the right, a small mineral sample is put into the flame as either a gas, sprayed solution, or on a small loop of wire, usually platinum. The flame evaporates the mineral and breaks chemical bonds to create free atoms. Each element emits light at a characteristic wavelength. These emissions are dispersed by a grating or prism and detected in the spectrometer.
If a Bunsen burner is available where you are trying to chemically analyze a mineral sample, inserting a small piece safely in the flame could prove helpful as the second image down on the right shows.
Symbol | Name | Color | Image |
---|---|---|---|
Al | Aluminium | Silver-white, in very hot such as an electric arc, light blue | File:Aluminum flame.png |
As | Arsenic | Blue | File:FlammenfärbungAs.jpg |
B | Boron | Bright green | File:FlammenfärbungB.png |
Ba | Barium | Pale/Apple green | File:Barium flame.png |
Be | Beryllium | White | |
Bi | Bismuth | Azure | |
Ca | Calcium | Orange | File:FlammenfärbungCa.png |
Cd | Cadmium | Brick red | |
Ce | Cerium | Blue | |
Co | Cobalt | Silver-white | |
Cr | Chromium | Silver-white | |
Cs | Caesium | Blue-Violet | File:Caesium flame.png |
Cu(I) | Copper(I) | Bluish-green | File:Copper (I) blue flame.png |
Cu(II) | Copper(II) (non-halide) | Green | Flame test on copper sulfate |
Cu(II) | Copper(II) (halide) | Blue-green | File:Cu+2 (CuCl2)-Blue.jpg |
Ge | Germanium | Pale blue | |
Fe(II) | Iron(II) | Gold, when very hot such as an electric arc, bright blue, or green turning to orange-brown | |
Fe(III) | Iron(III) | Orange-brown | An iron (III) flame, generated using the thermite reaction |
Hf | Hafnium | White | |
Hg | Mercury | Red | |
In | Indium | Indigo/Blue | |
K | Potassium | Lilac | File:Potassium flame.png |
Li | Lithium | crimson red; invisible through green glass | File:FlammenfärbungLi.png |
Mg | Magnesium | (none), but for burning Mg metal Intense White | File:Mg-flame.jpg |
Mn (II) | Manganese (II) | Yellowish green | |
Mo | Molybdenum | Yellowish green | |
Na | Sodium | Intense yellow; invisible through cobalt blue glass | ![]() |
Nb | Niobium | Green or blue | |
Ni | Nickel | Silver-white (sometimes reported as colorless) | |
P | Phosphorus | Pale bluish green | |
Pb | Lead | Blue/white | File:FlammenfärbungPb.png |
Ra | Radium | Crimson red | File:Radium flame.png |
Rb | Rubidium | Red-violet | File:Die Flammenfärbung des Rubidium.jpg |
Sb | Antimony | Pale green | File:FlammenfärbungSb.png |
Sc | Scandium | Orange | |
Se | Selenium | Azure blue | |
Sn | Tin | Blue-white | |
Sr | Strontium | Crimson to Scarlet, yellowish through green glass and violet through blue cobalt glass | File:Strontium flame.png |
Ta | Tantalum | Blue | |
Te | Tellurium | Pale green | |
Ti | Titanium | Silver-white | |
Tl | Thallium | Pure green | File:Thallium flame.png |
V | Vanadium | Yellowish Green | |
W | Tungsten | Green | |
Y | Yttrium | Carmine, Crimson, or Scarlet | |
Zn | Zinc | Colorless (sometimes reported as bluish-green) | File:Zinc burning.JPG |
Zr | Zirconium | Mild red |
From a flame analysis of a small mineral sample that easily dissolved in water, the chemical sodium was detected.
"Halide ions in solutions are detected using silver nitrate solutions. The test solution is acidified using a few drops of dilute nitric acid, and then a few drops of silver nitrate solution are added. Different coloured silver halide precipitates form, depending on the halide ions present:"[3]
- chloride ions give a white precipitate of silver chloride
- bromide ions give a cream precipitate of silver bromide
- iodide ions give a yellow precipitate of silver iodide
Fluorescences
Def. the "emission of light (or other electromagnetic radiation) by a material when stimulated by the absorption of radiation or of a subatomic particle"[4] is called fluorescence.
Fluorescence is the emission of light by a substance that has absorbed light or other electromagnetic radiation. It is a form of luminescence. In most cases, the emitted light has a longer wavelength, and therefore lower energy, than the absorbed radiation. However, when the absorbed electromagnetic radiation is intense, it is possible for one electron to absorb two photons; this two-photon absorption can lead to emission of radiation having a shorter wavelength than the absorbed radiation. The emitted radiation may also be of the same wavelength as the absorbed radiation, termed "resonance fluorescence".[5]
The most striking examples of fluorescence occur when the absorbed radiation is in the ultraviolet region of the spectrum, and thus invisible to the human eye, and the emitted light is in the visible region.
The common fluorescent lamp relies on fluorescence. Inside the glass tube is a partial vacuum and a small amount of mercury. An electric discharge in the tube causes the mercury atoms to emit ultraviolet light. The tube is lined with a coating of a fluorescent material, called the phosphor, which absorbs the ultraviolet and re-emits visible light. Fluorescent lighting is more energy-efficient than incandescent lighting elements. However, the uneven spectrum of traditional fluorescent lamps may cause certain colors to appear different than when illuminated by incandescent light or daylight. The mercury vapor emission spectrum is dominated by a short-wave UV line at 254 nm (which provides most of the energy to the phosphors), accompanied by visible light emission at 436 nm (blue), 546 nm (green) and 579 nm (yellow-orange). These three lines can be observed superimposed on the white continuum using a hand spectroscope, for light emitted by the usual white fluorescent tubes. These same visible lines, accompanied by the emission lines of trivalent europium and trivalent terbium, and further accompanied by the emission continuum of divalent europium in the blue region, comprise the more discontinuous light emission of the modern trichromatic phosphor systems used in many compact fluorescent lamp and traditional lamps where better color rendition is a goal.[6]
Reflectivity
Def. hue of a smooth surface of a mineral exhibited when sunlight reflects is called its color.
Def. the fraction of incident light or radiation reflected by a surface or body, commonly expressed as a percentage is called an albedo.
Def. reflectivity pertaining "to mirrors; mirror-like"[8] is called specular reflectivity.
Def. shine, "polish or sparkle"[9] is called luster, or lustre.
Def. the manner in which the surface of a mineral reflects light is called luster.
Def. having a shine, polish or sparkle comparable to resin is called a resinous luster, or is resinous.
Def. "in particular smooth and (partly) reflective"[10] is called vitreous, or glassy.
Def. "having a matte [diffuse] finish or no particular luster or brightness"[11] is called a dull luster.
Def. luster made "of, appearing to be made of, resembling, or related to metal"[12] is called a metallic luster.
Def. a luster, or reflectivity, "to maximize light return"[13] is called a brilliant luster.
Transmissivity
Def. the property that light passes through it almost undisturbed, such that one can see through it clearly is called transparency.
Def. the property that light passes through a mineral but detailed images do not is called translucency.
Def. not "allowing light to pass through"[14] is called opaque.
Neutrons
1. Nuclear reactor zones
2. Sandstone
3. Uranium ore layer
4. Granite. Credit: MesserWoland.
A natural nuclear fission reactor is a uranium mineral deposit where self-sustaining nuclear chain reactions have occurred. This can be examined by analysis of isotope ratios. The existence of this phenomenon was discovered in 1972 at Oklo in Gabon, Africa. Oklo is the only known location for this in the world and consists of 16 sites at which self-sustaining nuclear fission reactions took place approximately 1.7 billion years ago, and ran for a few hundred thousand years, averaging 100 kW of thermal power during that time.[15][16]
Gadolinium as a metal or salt has exceptionally high absorption of neutrons and therefore is used for shielding in neutron radiography and in nuclear reactors.
"The report by Hoffman et al. (1971) of 8.2 x 107 y 244Pu in terrestrial bastnesite is supported by some unpublished evidence at Argonne National Laboratory for 244Pu in terrestrial gadolinite (Metta et al., 1971)."[17]
The deep blue version of aquamarine is called maxixe. Maxixe is commonly found in the country of Madagascar. Its color fades to white when exposed to sunlight or is subjected to heat treatment, though the color returns with irradiation.
Dark-blue maxixe color can be produced in green, pink or yellow beryl by irradiating it with high-energy particles (gamma rays, neutrons or even X-rays).[18]
Beta particles
Excessive "26Mg [has] been reported in meteoritic carbonaceous chondrites [...] which demonstrate an excess of 26Mg of up to 40% combined with essentially solar concentrations of 24Mg and 25Mg. Many of the data are well correlated with the 27Al content of the samples, and this is interpreted as evidence that the excess 26Mg has arisen from the in situ decay (via positron emission and electron capture) of the ground state of 26Al in these minerals."[19]
Gamma rays
The peak at 40 keV is not from the mineral. From the color of the rock shown the yellowish mineral is likely to be autunite.
Autunite occurs as an oxidizing product of uranium minerals in granite pegmatites and hydrothermal deposits.
Ultraviolets
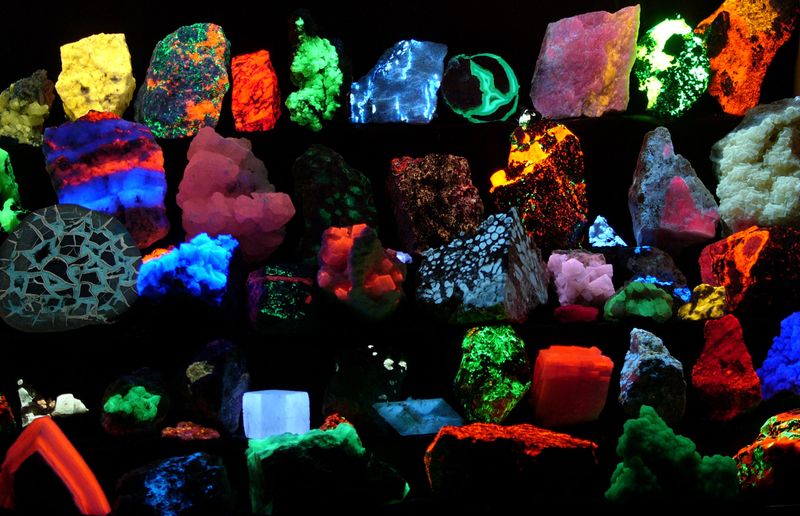
Ultraviolet lamps are also used in analyzing minerals and gems. Materials may look the same under visible light, but fluoresce to different degrees under ultraviolet light, or may fluoresce differently under short wave ultraviolet versus long wave ultraviolet.
Ultraviolet lamps may cause certain minerals to fluoresce, and is a key tool in prospecting for tungsten mineralisation.
Many samples of fluorite exhibit fluorescence under ultraviolet light, a property that takes its name from fluorite.[20] Many minerals, as well as other substances, fluoresce. Fluorescence involves the elevation of electron energy levels by quanta of ultraviolet light, followed by the progressive falling back of the electrons into their previous energy state, releasing quanta of visible light in the process. In fluorite, the visible light emitted is most commonly blue, but red, purple, yellow, green and white also occur. The fluorescence of fluorite may be due to mineral impurities such as yttrium, ytterbium, or organic matter in the crystal lattice. In particular, the blue fluorescence seen in fluorites from certain parts of Great Britain responsible for the naming of the phenomenon of fluorescence itself, has been attributed to the presence of inclusions of divalent europium in the crystal.[21]
"Between 190 and 1700 nm, the ordinary refractive index varies roughly between 1.9 and 1.5, while the extraordinary refractive index varies between 1.6 and 1.4.[22]
Under longwave (365 nm) ultraviolet light, diamond may fluoresce a blue, yellow, green, mauve, or red of varying intensity. The most common fluorescence is blue, and such stones may also phosphoresce yellow—this is thought to be a unique combination among gemstones. There is usually little if any response to shortwave ultraviolet.
Visuals

Beryl of various colors is found most commonly in granitic pegmatites, but also occurs in mica schists. Goshenite [a beryl clear to white cyclosilicate] is found to some extent in almost all beryl localities.
Quartzite (from German Quarzit[23]) is a hard, non-foliated metamorphic rock which was originally pure quartz sandstone.[24][25] Sandstone is converted into quartzite through heating and pressure usually related to tectonic compression within orogenic belts. Pure quartzite is usually white to gray, though quartzites often occur in various shades of pink and red due to varying amounts of iron oxide (Fe2O3). Other colors, such as yellow and orange, are due to other mineral impurities.
The great majority of silicates are oxides which comprise the majority of the earth's crust, as well as the other terrestrial planets, rocky moons, and asteroids. Sand, Portland cement, and thousands of minerals are examples of silicates.
Mineralogically, silicate minerals are divided according to structure of their silicate anion into the following groups:[26][27]
- Nesosilicates (lone tetrahedron) - [SiO4]4−, e.g. olivine, tephroite.
- Sorosilicates (double tetrahedra) - [Si2O7]6−, e.g. epidote, melilite group, leucophanite.
- Cyclosilicates (rings) - [SinO3n]2n−, e.g. tourmaline group.
- Inosilicates (single chain) - [SinO3n]2n−, e.g. pyroxene group.
- Inosilicates(double chain) - [Si4nO11n]6n−, e.g. amphibole group.
- Phyllosilicates (sheets) - [Si2nO5n]2n−, e.g. micas and clays like Kaolin.
- Tectosilicates (3D framework) - [AlxSiyO2(x+y)]x−, e.g. quartz, feldspars, zeolites.
Violets
Axinite-(Mg) or magnesioaxinite, Ca2MgAl2BOSi4O15(OH) magnesium rich, [can be] pale blue to pale violet[28]
Fluorapatite a sample of which is shown at right is a mineral with the formula Ca5(PO4)3F (calcium fluorophosphate). Fluorapatite as a mineral is the most common phosphate mineral. It occurs widely as an accessory mineral in igneous rocks and in calcium rich metamorphic rocks. It commonly occurs as a detrital or diagenic mineral in sedimentary rocks and is an essential component of phosphorite ore deposits. It occurs as a residual mineral in lateritic soils.[29]
At lower left is another fluorapatite example that is violet in color on quartz crystals.
Lower right shows both a rough stone and a cut stone of tanzanite. "Tanzanite is the blue/purple variety of the mineral zoisite (a calcium aluminium hydroxy silicate) with the formula (Ca2Al3(SiO4)(Si2O7)O(OH))]. Tanzanite is noted for its remarkably strong trichroism, appearing alternately sapphire blue, violet and burgundy depending on crystal orientation.[30] Tanzanite can also appear differently when viewed under alternate lighting conditions. The blues appear more evident when subjected to fluorescent light and the violet hues can be seen readily when viewed under incandescent illumination. A rough violet sample of tanzanite is third down at left.
Tanzanite in its rough state is usually a reddish brown color. It requires artificial heat treatment to 600 °C in a gemological oven to bring out the blue violet of the stone.[31]
Tanzanite is found only in the foothills of Mount Kilimanjaro.
Tanzanite is universally heat treated in a furnace, with a temperature between 550 and 700 degrees Celsius, to produce a range of hues between bluish-violet to violetish-blue. Some stones found close to the surface in the early days of the discovery were gem-quality blue without the need for heat treatment.
Perhaps the most common violet mineral is sapphire. A sample of uncut natural sapphire is at lowest right. "Sapphires may be found naturally, by searching through certain sediments (due to their resistance to being eroded compared to softer stones) or rock formations.
Lepidolite (KLi2Al(Al,Si)3O10(F,OH)2 is a lilac-gray or rose-colored member of the mica group that is a secondary source of lithium. It is a phyllosilicate mineral[27]
It is associated with other lithium-bearing minerals like spodumene in pegmatite bodies. It is one of the major sources of the rare alkali metals rubidium and caesium.[32]
It occurs in granite pegmatites, in some high-temperature quartz veins, greisens and granites. Associated minerals include quartz, feldspar, spodumene, amblygonite, tourmaline, columbite, cassiterite, topaz and beryl.[29]
Blues
Usually, Hibonite ((Ca,Ce)(Al,Ti,Mg)12O19) as shown at right is a brownish black mineral. It is rare, but is found in high-grade metamorphic rocks on Madagascar. Some presolar grains in primitive meteorites consist of hibonite. Hibonite also is a common mineral in the Ca-Al-rich inclusions (CAIs) found in some chondrite chondritic meteorites. Hibonite is closely related to hibonite-Fe (IMA 2009-027, ((Fe,Mg)Al12O19)) an alteration mineral from the Allende meteorite.[33] Hibonite is blue perhaps like the image at left in meteorite occurrence. Often a mineral appears blue due to the presence of copper or sulfur. Glaucophane is a blue silicate that owes its color to its characteristic formation.
Sodalite
Sodalite is a rich royal blue mineral massive sodalite samples are opaque, crystals are usually transparent to translucent. Occurring typically in massive form, sodalite is found as vein fillings in plutonic igneous rocks such as nepheline syenites.
Covellite
Covellite has been found in veins at depths of 1,150 meters, as the primary mineral. Covellite formed as clusters in these veins reaching one meter across.
Lazurite
Lazurite is a tectosilicate mineral with sulfate, sulfur and chloride with formula: (Na,Ca)8[(S,Cl,SO4,OH)2|(Al6Si6O24)]. It is a feldspathoid and a member of the sodalite group. The colour is due to the presence of S3- anions. Lazurite is a product of contact metamorphism of limestone.
Blueschist facies
Template:Metamorphic facies to click |
Diagram showing metamorphic facies in pressure-temperature space. The domain of the graph corresponds to circumstances within the Earth's crust and upper mantle. |
A metamorphic facies is a set of metamorphic mineral assemblages that were formed under similar pressures and temperatures.[34] The assemblage is typical of what is formed in conditions corresponding to an area on the two dimensional graph of temperature vs. pressure (See diagram at right).[34] Rocks which contain certain minerals can therefore be linked to certain tectonic settings, times and places in geological history of the area.[34] The boundaries between facies (and corresponding areas on the temperature v. pressure graph), are wide, because they are gradational and approximate.[34] The area on the graph corresponding to rock formation at the lowest values of temperature and pressure, is the range of formation of sedimentary rocks, as opposed to metamorphic rocks, in a process called diagenesis.[34]
Blueschist is a metavolcanic rock that forms by the metamorphism of basalt and rocks with similar composition at high pressures and low temperatures, approximately corresponding to a depth of 15 to 30 kilometers and 200 to ~500 degrees Celsius. The blue color of the rock comes from the presence of the mineral glaucophane. Blueschists are typically found within orogenic belts as terranes of lithology in faulted contact with greenschist or rarely eclogite facies rocks. ... Blueschist, as a rock type, is defined by the presence of the minerals glaucophane + ( lawsonite or epidote ) +/- jadeite +/- albite or chlorite +/- garnet +/- muscovite in a rock of roughly basaltic composition. Blueschist often has a lepidoblastic, nematoblastic or schistose rock microstructure defined primarily by chlorite, phengitic white mica, glaucophane, and other minerals with an elongate or platy shape. Grain size is rarely coarse, as mineral growth is retarded by the swiftness of the rock's metamorphic trajectory and perhaps more importantly, the low temperatures of metamorphism and in many cases the anhydrous state of the basalts. However, coarse varieties do occur. Blueschists may appear blue, black, gray, or blue-green in outcrop.
Glaucophane
Glaucophane is a mineral belonging to the amphibole group, chemical formula Na2Mg3Al2Si8O22(OH)2. The blue color is very diagnostic for this species. It, along with the closely related mineral riebeckite are the only common amphibole minerals that are typically blue. Glaucophane forms in metamorphic rocks that are either particularly rich in sodium or that have experienced low temperature-high pressure metamorphism such as would occur along a subduction zone. This material has undergone intense pressure and moderate heat as it was subducted downward toward the mantle. It is glaucophane's color that gives the blueschist facies its name. Glaucophane is also found in eclogites that have undergone retrograde metamorphism.[35]
Hauyne
Hauyne, haüyne or hauynite [occurs] in Vesuvian lavas in Monte Somma, Italy,[36] ... It is a tectosilicate mineral with sulfate, with endmember formula Na3Ca(Si3Al3)O12(SO4).[37] ... It is a feldspathoid and a member of the sodalite group.[38][39] Haüyne occurs in phonolites and related leucite- or nepheline-rich, silica-poor, igneous rocks; less commonly in nepheline-free extrusives[40][38][39][41] and metamorphic rocks (marble).[38]
Water ice
Blue ice occurs when snow falls on a glacier, is compressed, and becomes part of a glacier blue ice was observed in Tasman Glacier, New Zealand in January 2011.[42] Ice is blue for the same reason water is blue: it is a result of an overtone of an oxygen-hydrogen (O-H) bond stretch in water which absorbs light at the red end of the visible spectrum.[43]
Cyans
The gem-gravel placer deposits of Sri Lanka contain aquamarine.
The deep blue version of aquamarine is called maxixe. Maxixe is commonly found in the country of Madagascar. Its color fades to white when exposed to sunlight or is subjected to heat treatment, though the color returns with irradiation.
"The pale blue color of aquamarine is attributed to Fe2+. The Fe3+ ions produce golden-yellow color, and when both Fe2+ and Fe3+ are present, the color is a darker blue as in maxixe. Decoloration of maxixe by light or heat thus may be due to the charge transfer Fe3+ and Fe2+.[44][45][46][47] Dark-blue maxixe color can be produced in green, pink or yellow beryl by irradiating it with high-energy particles (gamma rays, neutrons or even X-rays).[18]
Turquoise at right is an opaque, blue-to-green mineral that is a hydrous phosphate of copper and aluminium, with the chemical formula CuAl6(PO4)4(OH)8·4H2O.
Although fluorite usually appears violet or purple in color, the crystals at left are cyan with some blue or violet fluorite mixed in suggesting slight variations in composition.
Greens
Green is the color of emeralds and jade.[49]
"Olivines are described by Mg2yFe2-2ySiO4, with y in the range [0, 1]."[50] Substituting values for y from 0 to 1 produce ideal compositions from forsterite Mg2SiO4 to fayalite Fe2SiO4.
"Laboratory studies of the evolution of a magnesium silicate smoke from an amorphous condensate to a crystalline mineral by annealing in vacuum provide a foundation for the development of a silicate evolution index (SEI)."[51]
Yellows
Carnotites
Carnotite is a potassium uranium vanadate radioactive mineral with chemical formula: K2(UO2)2(VO4)2·3H2O. The water content can vary and small amounts of calcium, barium, magnesium, iron, and sodium are often present. ... Carnotite is a bright to greenish yellow mineral that occurs typically as crusts and flakes in sandstones. Amounts as low as one percent will color the sandstone a bright yellow. The high uranium content makes carnotite an important uranium ore and also radioactive. It is a secondary vanadium and uranium mineral usually found in sedimentary rocks in arid climates. It is an important ore of uranium in the Colorado Plateau region of the United States where it occurs as disseminations in sandstone and concentrations around petrified logs.
Cubanites
Cubanite is a yellow mineral of copper, iron, and sulfur, CuFe2S3.[52] Cubanite occurs in high temperature hydrothermal deposits with pyrrhotite and pentlandite as intergrowths with chalcopyrite. It results from exsolution from chalcopyrite at temperatures below 200 to 210 °C.[53] It has also been reported from carbonaceous chondrite meteorites.[53]
Golds
At right is an image of a piece of native gold discovered as part of a placer deposit, a gold nugget.
Limonites
Limonite is an iron ore consisting of a mixture of hydrated iron(III) oxide-hydroxides in varying composition. The generic formula is frequently written as FeO(OH)·nH2O, although this is not entirely accurate as the ratio of oxide to hydroxide can vary quite widely. Limonite is one of the two principle iron ores, the other being hematite, and has been mined for the production of iron since at least 2500 BCE.[54][55] Although originally defined as a single mineral, limonite is now recognized as a mixture of related hydrated iron oxide minerals, among them goethite, akaganeite, lepidocrocite, and jarosite. Individual minerals in limonite may form crystals, but limonite does not, although specimens may show a fibrous or microcrystalline structure,[56] and limonite often occurs in concretionary forms or in compact and earthy masses; sometimes mammillary, botryoidal, reniform or stalactitic. Because of its amorphous nature, and occurrence in hydrated areas limonite often presents as a clay or mudstone. However there are limonite pseudomorphs after other minerals such as pyrite.[57] This means that chemical weathering transforms the crystals of pyrite into limonite by hydrating the molecules, but the external shape of the pyrite crystal remains. Limonite pseudomorphs have also been formed from other iron oxides, hematite and magnetite; from the carbonate siderite and from iron rich silicates such as almandine garnets. Limonite usually forms from the hydration of hematite and magnetite, from the oxidation and hydration of iron rich sulfide minerals, and chemical weathering of other iron rich minerals such as olivine, pyroxene, amphibole, and biotite. It is often the major iron component in lateritic soils. One of the first uses was as a pigment. The yellow form produced yellow ochre for which Cyprus was famous.[58]
Microlites
Microlite is composed of sodium calcium tantalum oxide with a small amount of fluorine (Na,Ca)2Ta2O6(O,OH,F). Microlite is a mineral in the pyrochlore group that occurs in pegmatites and constitutes an ore of tantalum. It has a Mohs hardness of 5.5 and a variable specific gravity of 4.2 to 6.4. It occurs as disseminated microscopic subtranslucent to opaque octahedral crystals with a refractive index of 2.0 to 2.2. Microlite is also called djalmaite. Microlite occurs as a primary mineral in lithium-bearing granite pegmatites, and in miarolitic cavities in granites.
Orpiments
Orpiment, [Arsenic trisulfide] As2S3, is a common monoclinic arsenic sulfide mineral. Orpiment is an orange to yellow mineral that is found worldwide [on Earth], and occurs as a sublimation product in volcanic fumaroles, low temperature hydrothermal veins, hot springs and as a byproduct of the decay of another arsenic mineral, realgar.
Pyrites
The mineral pyrite, or iron pyrite, is an iron sulfide with the formula FeS2. This mineral's metallic luster and pale brass-yellow hue have earned it the nickname fool's gold because of its superficial resemblance to gold. Pyrite is the most common of the sulfide minerals [on Earth]. Pyrite is usually found associated with other sulfides or oxides in quartz veins, sedimentary rock, and metamorphic rock, as well as in coal beds, and as a replacement mineral in fossils. Despite being nicknamed fool's gold, pyrite is sometimes found in association with small quantities of gold. Gold and arsenic occur as a coupled substitution in the pyrite structure. In the Carlin–type gold deposits, arsenian pyrite contains up to 0.37 wt% gold.[59]
Satterlyites
Satterlyite is a hydroxyl bearing iron phosphate mineral. The mineral can be found in phosphetic shales. Satterlyite is part of the phosphate mineral group. Satterlyite is a transparent, light brown to light yellow mineral. Satterlyite has a formula of (Fe2+,Mg,Fe3+)2(PO4)(OH). Satterlyite occurs in nodules in shale in the Big Fish River (Mandarino, 1978). These nodules were about 10 cm in diameter, some would consist of satterlyite only and others would show satterlyite with quartz, pyrite, wolfeite or maricite.
"Holtedahlite, a mineral that was found in Tingelstadtjern quarry in Norway, with the formula (Mg12PO4)5(PO3OH,CO3)(OH,O)6 is isostructural with satterlyite (Raade, 1979). Infrared absorption powder spectra show that satterlyite is different than natural haltedahlite in that there is no carbonate for phosphate substitution (Kolitsch, 2002). Satterlyite is also structurally related to phosphoellenbergerite, a mineral that was discovered in Modum, Norway; near San Giocomo Vallone Di Gilba, in Western Alps of Italy (Palache, 1951); the minerals formula is Mg14(PO4)5(PO3OH)2(OH)6 (Kolitsch, 2002).
Spurrites
Spurrite is a nesosilicate that can occur naturally as a yellow mineral. "Its chemical formula is Ca5(SiO4)2CO3.[60] Spurrite is generally formed in contact metamorphism zones as mafic magmas are intruded into carbonate rocks.[61]
Sulfurs
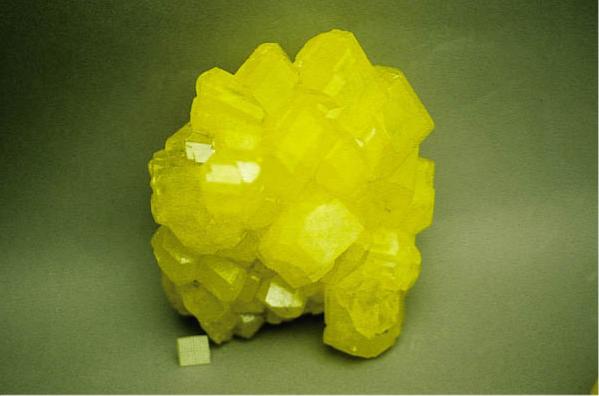
Sulfur occurs naturally as the pure element (native sulfur) and as sulfide and sulfate minerals. Being abundant in native form, sulfur was known in ancient times, mentioned for its uses in ancient India, ancient Greece, China and Egypt. Octasulfur is a soft, bright-yellow solid with only a faint odor, similar to that of matches.
Oranges
The mineral orpiment [at top right] is a source of yellow and orange pigments. Realgar is an arsenic sulfide mineral of 1.5-2.5 Mohs hardness is used to make red-orange pigment. Crocoite crystals from Dundas extended mine in Tasmania is used to make the first synthetic orange pigment, chrome orange.
Calcite [at second left] is a common calcium carbonate mineral that occurs in orange.
Reds
Rhodolite is a varietal name for rose-pink to red mineral pyrope, a species in the garnet group.
Chemically, rhodolite is an iron-magnesium-aluminium silicate, [(Mg,Fe)3Al2(SiO4)3,] part of the pyrope-almandine solid-solution series, with an approximate garnet composition of Py70Al30.
Breithauptite is a nickel antimonide mineral with the simple formula NiSb. Breithauptite is a metallic opaque copper-red mineral crystallizing in the hexagonal - dihexagonal dipyramidal crystal system. It is typically massive to reniform in habit, but is observed as tabular crystals. It has a Mohs hardness of 3.5 to 4 and a specific gravity of 8.23.
It occurs in hydrothermal calcite veins associated with cobalt–nickel–silver ores.
Cinnabar or cinnabarite (red mercury(II) sulfide (HgS), native vermilion), is the common ore of mercury. Its color is cochineal-red, towards brownish red and lead-gray. Cinnabar may be found in a massive, granular or earthy form and is bright scarlet to brick-red in color.[62] Generally cinnabar occurs as a vein-filling mineral associated with recent volcanic activity and alkaline hot springs. Cinnabar is deposited by epithermal ascending aqueous solutions (those near surface and not too hot) far removed from their igneous source.
Crocoite is a mineral consisting of lead chromate, PbCrO4. Crystals are of a bright hyacinth-red color. Relative rarity of crocoite is connected with specific conditions required for its formation: an oxidation zone of lead ore bed and presence of ultramafic rocks serving as the source of chromium (in chromite).
Eudialyte is a somewhat rare, red silicate mineral, which forms in alkaline igneous rocks, such as nepheline syenites.
Hematite is the mineral form of iron(III) oxide (Fe2O3), one of several iron oxides. Hematite is colored black to steel or silver-gray, brown to reddish brown, or red. Huge deposits of hematite are found in banded iron formations.
Infrareds
"On October 7, 2009, the presence of water ice was confirmed on the surface of [24 Themis] using NASA’s Infrared Telescope Facility. The surface of the asteroid appears completely covered in ice. As this ice layer is sublimated, it may be getting replenished by a reservoir of ice under the surface. Organic compounds were also detected on the surface.[63][64][65][66]
Trace amounts of water would be continuously produced by high-energy solar protons impinging oxide minerals present at the surface of the asteroid. The hydroxyl surface groups (S–OH) formed by the collision of protons (H+) with oxygen atoms present at oxide surface (S=O) can further be converted in water molecules (H2O) adsorbed onto the oxide minerals surface. The chemical rearrangement supposed at the oxide surface could be schematically written as follows:
- 2 S-OH → S=O + S + H2O
or,
- 2 S-OH → S–O–S + H2O
where S represents the oxide surface.[67]
Submillimeters
"Optical constants of natural minerals [using millimeter and submillimeter wavelength spectroscopy] are of interest for characterizing interstellar dust, for remote sensing of terrain and for light scattering in the atmosphere by soil particles."[68]
Hydrogens
"Oxygen and hydrogen isotope analyses have been made on a variety of clay minerals of sedimentary and diagenetic origins. The interlayer water of clay minerals was found to exchange rapidly with atmospheric water. Conditions under which the interlayer water could be removed from the clays without affecting the isotopic compositions of their aluminosilicate oxygen and hydrogen were therefore determined, and the interlayer water was routinely removed and discarded prior to isotopic analysis."[69]
Magnesiums
{{free media}}
At right is a visual close up of green sand which is actually olivine crystals that have been eroded from lava rocks. Some olivine crystals are still inside the lava rock.
Forsterite (Mg2SiO4) is the magnesium rich end-member of the olivine solid solution series.
Forsterite is associated with igneous and metamorphic rocks and has also been found in meteorites. In 2005 it was also found in cometary dust returned by the Stardust probe.[70] In 2011 it was observed as tiny crystals in the dusty clouds of gas around a forming star.[71]
Two polymorphs of forsterite are known: wadsleyite (also orthorhombic) and ringwoodite (isometric). Both are mainly known from meteorites.
At lower right is an image of a small volcanic bomb of (black) basanite with (green) dunite.
Dunite is an igneous, plutonic rock, of ultramafic composition, with coarse-grained or phaneritic texture. The mineral assemblage is greater than 90% olivine, with minor amounts of other minerals such as pyroxene, chromite and pyrope. Dunite is the olivine-rich end-member of the peridotite group of mantle-derived rocks. Dunite and other peridotite rocks are considered the major constituents of the Earth's mantle above a depth of about 400 kilometers. Dunite is rarely found within continental rocks, but where it is found, it typically occurs at the base of ophiolite sequences where slabs of mantle rock from a subduction zone have been thrust onto continental crust by obduction during continental or island arc collisions (orogeny). It is also found in alpine peridotite massifs that represent slivers of sub-continental mantle exposed during collisional orogeny. Dunite typically undergoes retrograde metamorphism in near-surface environments and is altered to serpentinite and soapstone.
Magnesium (Mg I) has an absorption band at 416.727±2.9 nm with an excitation potential of 4.33 eV.[72]
Magnesium (Mg II) has an absorption band at 439.059±6.6 nm with an excitation potential of 9.96 eV.[72]
"Glaciers in the Karakoram and western Himalaya (site 2 and 3) show high annual snow accumulation rates and high annual fluxes of calcium, sodium, chloride, sulfate, and nitrate."[73]
Aluminums
{{free media}}
{{free media}}
"The aluminium abundance was derived from the resonance line at 394.4nm, and Al is underabundant by ∼ −0.7 dex with respect to iron."[74] "These abundances are the LTE values; no NLTE corrections, as prescribed by Baumüller and Gehren (1997) and Baumüller et al. (1998), have been applied. The prescribed NLTE corrections for Teff = 6500K, log g = 4.0, [Fe/H] = –3.0 are –0.11 ... for ... Al .... If we assume these values to apply for our lower-gravity star [CS 29497-030], then Al follows iron"[74]. The elemental abundance ratios for CS 29497-030 of aluminum are [Al/H] = -3.37, [Al/Fe] = -0.67.[74]
Both Al I absorption lines at 394.401±8.5 and 396.152±6.5 have been measured for Sirius.[72]
"As Andean glaciers recede, there has been an increase in seasonal discharge and in catchments with the least glacierized area and a decrease in total annual discharge [...] Dry season examinations, including this study, are particularly important because during this period glacial melt provides up to 40% of the total discharge in the Cordillera Blanca (Mark et al., 2005). The dry season thus provides the greatest potential opportunity to evaluate water quality deterioration related to glacial retreat. [...] In the Cordillera Blanca, the exposure of fresh sulfide-rich lithologies by retreating glaciers (Wilson et al., 1967) is thus integral to the biogeochemistry of proglacial streams. [...] the dry season geochemistry of trace and minor elements was examined in the proglacial Rio Quilcay from within 1 km of its glacier origins to 12 km downstream."[75]
The "Rio Quilcay [is] a glacial-fed tributary to the Upper Rio Santa in the uppermost 12 km at elevations ranging from approximately 4800 to ~3800 m.a.s.l. [...] The sampled region of the Rio Quilcay receives glacial melt directly and indirectly from two proglacial lakes: Cuchillacocha and Tulpacocha. Geology in this region of the Cordillera Blanca includes pyrite schists and phyllite and pyrite-bearing quartzite intruded by a central granodiorite-tonalite batholith all overlain by clastic sediments deposited during glacial retreat (Wilson et al., 1967). Sulfide-rich lithologies are prevalent especially in the north-eastern high-altitude regions of the Cordillera Blanca (e.g. the Rio Quilcay Valley) with fresh exposures resulting from glacial scour (Wilson et al., 1967). Many headwaters in the Cordillera Blanca, including the Rio Quilcay and its tributaries, have ochreous precipitates"[75]
"Aluminum, Ca, Fe, K, Mn, Na, and Si were determined using an Optima 3000 DV Inductively Coupled Plasma-Optical Emission Spectrometer (ICP-OES) using five calibration standards that bracketed the range of concentrations within the samples, excepting the three highest samples which were diluted 1:10 before analyses. Cobalt, Cu, Ni, Pb and Zn were determined on a Perkin–Elmer Sciex Elan 6000Ⓡ Inductively Coupled Plasma-Mass Spectromenter (ICP-MS) also using five calibration standards, however with no sample dilution. All element results were drift corrected. Sulfate and NO3- were determined using a Dionex DX-120 ion chromatograph (IC). Only SO42- is reported because other anions fall near detections limits (DLs) in the higher elevation samples, or represent less than 5% of the charge balance in the pH < 4 streams."[75]
It "is likely that both the sulfide-rich lithology underlying the Rio Quilcay and the near-glacier sample locations enhanced sulfide weathering, and generated exceptionally high cation loads."[75]
"Elevated dissolved Al, Fe and Cu concentrations (6.1mg/L, 21.4 mg/L, 6.1 lg/L) were observed at site 11, 0.3 km immediately downstream of a moraine. Concentrations of these elements increased by more than four times the concentrations at site 10. Concentration gains were likely associated with glacier melt rapidly weathering minerals within the moraine (Brown, 2002)."[75]
"Tributary C also influenced the chemical composition of the stream immediately below its inflow at site 13. In fact, Fe reached the second highest concentration reported (12.8 mg/L) and dissolved Al, Mn, Co, Cu, Ni and Zn concentrations also increased above their upstream values. Tributary C overlays a region with enhanced sulfide mineral oxidation [image at the right]. Evidence for this includes a major cation: SO42- equivalent ratio of 1, and abundant algal mats covered with yellow and orange precipitates (Bigham et al., 1996). In addition, dissolved Al and Zn increased an additional 270% and 160% relative to site 13–14, respectively, and after the inflow of tributary D."[75]
Calciums
{{free media}}
Calcium (Ca) has green emission lines at 526.170 nm and 526.556 nm as observed in solar limb faculae.[76]
As of 1977, "model calculations cannot reproduce the observed breadth of the Ca II λ3933 line in Da,F stars like Ross 627 without appealing to an unknown line-broadening mechanism".[77]
Calcium has a line occurring in the solar corona at 408.63 nm of Ca XIII.[78]
Calcium (Ca I) has two absorption bands, 422.673±4.5 nm and 430.253±0.6.[72] The second has an excitation potential of 1.89 eV.[72]
Calcium (Ca II) has an absorption band, 393.366±55.0.[72]
"Heinrich Layers are found in the North Atlantic Ocean as well-constrained markers of catastrophic iceberg surges from the Pan-Atlantic ice sheets during the last glacial cycle. Their physical and geochemical characteristics [...] are predominantly due to the source sediments of the ice-rafted debris (IRD) on the one hand (magnetic susceptibility, color, carbonate content) and the response of the palaeo-environment on the other hand (carbonate content, foraminiferal assemblage)."[79]
"Sediment cores in the Porcupine Seabight (West off Ireland) have shown the presence of Heinrich Events without the diagnostic changes in magnetic susceptibility (MS) [...] the concentration of ice-rafted debris (commonly referred to as the fraction > 150 μm) increases towards the culmination of HL2, marked by an increase in MS, [X-ray fluorescence] XRF Ca and the percentage of N. pachyderma s."[79]
The "zone where the density increases is marked by a cloud of fine and highly dense particles surrounding the IRD. [The] fine clayey “background” matrix throughout the core [consists] of zoned dolomites. [...] the mineralogical analyses [suggest] a predominant volcanic source for the magnetic susceptibility. [Both] XRF Fe and Ti show significant decreases near the HL culmination".[79]
Titaniums
{{free media}}
{{free media}}
Titanium (Ti) has green emission lines at 521.97, 522.268, 522.413, 524.729, and 526.596 nm as observed in solar limb faculae.[76]
Titanium (Ti II) has an absorption band, 391.346-441.108 nm, with an excitation potential range of 0.60-3.08 eV.[72]
Titanium has two emission lines at 456.3757 and 457.1971 nm from Ti II.[80]
"Till collected from surface exposures within the valley of the Hudson River south of the Sanford Hill magnetite-ilmenite ore deposit in the Adirondack Mountains of New York is composed primarily of plagioclase, pyroxene, garnet, magnetite, and ilmenite [FeTiO3]. The concentration of magnetite and ilmenite in bulk till both decrease exponentially with increasing distance south of the ore bodies because of dilution by the entrainment of plagioclase and garnet + pyroxene from the bedrock and from older till deposits in the valley. Evidence for comminution of magnetite and ilmenite is provided by decreasing abundances of these minerals in the coarse fractions (1000-125 µm) and corresponding increases in the fine fraction (<125 µm). The apparent rate of comminution of ilmenite as a function of transport distance is significantly greater than that of magnetite, which causes ilmenite to be concentrated in the fine fraction of till compared to magnetite."[81]
Isotope geochemistry
A table of nuclides or chart of nuclides is a two-dimensional graph in which one axis represents the number of neutrons and the other represents the number of protons in an atomic nucleus. Each point plotted on the graph thus represents the nuclide of a real or hypothetical chemical element. Hydrogen is at the lower left.
Isotope geochemistry involves the determination of the relative and absolute concentrations of the [chemical] elements and their isotopes in the earth and on earth's surface.
For most stable isotopes, the magnitude of fractionation from kinetic and equilibrium fractionation is very small; for this reason, enrichments are typically reported in "per mil" (‰, parts per thousand).[82]
Enrichments (<math>\delta</math>) represent the ratio of heavy isotope to light isotope in the sample over the ratio of a standard.
- <math>\delta ^{A}isotope = \Biggl( \frac{\bigl( \frac{^{A}isotope}{^{B}isotope} \bigr)_{sample}}{\bigl( \frac{^{A}isotope}{^{B}isotope} \bigr)_{standard}} -1 \Biggr) * 1000\ ^{o}\!/\!_{oo}</math>
"The depletion of total [boron] B [in the Victorian volcanic-crater lakes of southeastern Australia] and the high positive δ 11B values relative to seawater (B/Cl ratio = 7.9 x 10-4; δ 11B = 39%.) are attributed to a marine (cyclic) salt origin together with adsorption processes in closed systems with low water/sediment (W/R) ratios."[83]
"Although the δ [11B] value of borate minerals may be a discriminant of marine or non-marine origin, boron isotopes are less distinctive in evaporative environments where boron is not an abundant component and where water/sediment interaction occurs."[83]
The incidence of 18O (the heavy isotope of oxygen) can be used as an indicator of polar ice sheet extent, and boron isotopes are key indicators of the pH and CO2 content of oceans in the geologic past.
Although rubidium is monoisotopic, naturally occurring rubidium is composed of two isotopes: the stable 85Rb (72.2%) and the radioactive 87Rb (27.8%).[84] Natural rubidium is radioactive with specific activity of about 670 (Becquerel) Bq/g, enough to significantly expose a photographic film in 110 days.[85][86]
Photogeochemistry
Photogeochemistry is the study of light-induced chemical reactions that occur or may occur among natural components of the earth's surface.[87]
If a certain compound is produced by an organism, and the organism dies but the compound remains, this compound may still participate independently in a photogeochemical reaction even though its origin is biological (e.g. biogenic mineral precipitates[88][89] or organic compounds released from plants into water[90]
The "inorganic colloid must possess the property of transforming sunlight, or some other form of radiant energy, into chemical energy."[91]
Many naturally occurring minerals are semiconductors that absorb some portion of solar radiation.[92]
Semiconducting minerals with appropriate band gaps and appropriate band energy levels can catalyze a vast array of reactions,[93] most commonly at mineral-water or mineral-gas interfaces.
Mercury
"The crater in the lower right-hand corner of this image [on the right] has a patch of very dark material located near its center. The region of this image has been seen only with the Sun high overhead in the sky. Such lighting conditions are good for recognizing color differences of rocks but not well suited for ascertaining the topography of surface features from shadows. The shape of the surface in this area is difficult to resolve given the lighting angle, but the dark patch is not in shadow. Dark surfaces have also been seen on other regions of Mercury, including this dark halo imaged during the second Mercury flyby (PIA11357) and near such named craters as Nawahi, Atget, and Basho seen during MESSENGER's first Mercury encounter. The example here is particularly striking, however, and from this NAC image the material may appear even darker than in other example areas. The dark color is likely due to rocks that have a different mineralogical composition from that of the surrounding surface. Understanding why these patches of dark rocks are found on Mercury's surface is a question of interest to the MESSENGER Science Team. The right edge of the image here aligns with this previously released NAC image (see PIA11763), where other dark surface material, as well as patches of light-colored rocks, can be seen."[94]
The second lower image on the right from the top shows "a double-ring impact basin, with another large impact crater on its south-south-western side. Double-ring basins are formed naturally when a large meteoroid strikes the surface of a rocky planet. Smaller, more recent impacts also formed comparatively fresh craters across the entire surface visible in this image. The floor within the inner or peak ring appears to be smoother than the floor between the peak ring and the outer rim, possibly the result of lava flows that partially flooded the basin some time after impact."[95]
The lowest image from the top on the right is a closeup of the weird terrain of Mercury.
"Weird terrain best describes this hilly, lineated region of Mercury. Scientists note that this area is at the antipodal point to the large Caloris basin. The shock wave produced by the Caloris impact may have been reflected and focused to the antipodal point, thus jumbling the crust and breaking it into a series of complex blocks. The area covered is about 800 km (497 mi) on a side."[96]
"One of the most prominent lobate scarps (Discovery Scarp) [on the left], photographed by Mariner 10 during it's first encounter with Mercury, is located at the center of this image (extending from the top to near bottom). This scarp is about 350 kilometers long and transects two craters 35 and 55 kilometers in diameter. The maximum height of the scarp south of the 55-kilometer crater is about 3 kilometers. Notice the shallow older crater (near the center of the image) perched on the crest of the scarp."[97]
"The Mariner 10 mission [...] explored Venus in February 1974 on the way to three encounters with Mercury-in March and September 1974 and in March 1975."[97]
"Optical reflectance studies of Mercury provide evidence for Mg silicates."[98]
The MESSENGER X-ray spectrometer (XRS) maps mineral composition within the top millimeter of the surface on Mercury by detecting X-ray spectral lines from magnesium, aluminum, sulphur, calcium, titanium, and iron, in the 1-10 keV range.[99][100]
Earth
Evidence from geoseismology, heat flow at the surface, and mineral physics is combined with the Earth's mass and moment of inertia to infer models of the Earth's interior - its composition, density, temperature, pressure. For example, the Earth's mean specific gravity (5.515) is far higher than the typical specific gravity of rocks at the surface (2.7–3.3), implying that the deeper material is denser. This is also implied by its low moment of inertia (0.33 M R2, compared to 0.4 M R2 for a sphere of constant density). However, some of the density increase is compression under the enormous pressures inside the Earth. The effect of pressure can be calculated using the Adams–Williamson equation. The conclusion is that pressure alone cannot account for the increase in density.
Reconstruction of seismic reflections in the deep interior indicate some major discontinuities in seismic velocities that demarcate the major zones of the Earth: inner core, outer core, mantle, lithosphere and crust.
The seismic model of the Earth does not by itself determine the composition of the layers. For a complete model of the Earth, mineral physics is needed to interpret seismic velocities in terms of composition. The mineral properties are temperature-dependent, so the geotherm must also be determined. This requires physical theory for thermal conduction and convection and the heat contribution of [radionuclides] radioactive elements. The main model for the radial structure of the interior of the Earth is the Preliminary Reference Earth Model (PREM). Some parts of this model have been updated by recent findings in mineral physics (see post-perovskite) and supplemented by seismic tomography.
Inner cores
We know that the Earth's core is composed of an alloy of iron and other minerals.[101]
"A PKJKP [P wave, traversing the outer core K, and the inner core J, to emerge again as the P wave] traverses the inner core as a shear wave, so this is the direct evidence that the inner core is solid, because only in the solid material the shear wave can exist. In the liquid material, say water, only the compressional wave can travel through."[102]
Studying "archived data from about 20 large earthquakes, all monitored by an array of German seismic detectors back in the 1980s and '90s" has "reliably detected" a PKJKP wave in 2005, demonstrating that the inner core is solid.[103]
The inner core, however, is solid because of the enormous pressure.[104]
The inner core "is a solid ball of superhot iron and nickel alloy about 760 miles (1,220 kilometers) in diameter. ... the inner core is, at 10,800 degrees Fahrenheit (6,000 degrees Celsius), as hot as the surface of the sun."[105]
"We know the Earth's inner core is composed mostly of iron".[106]
"The metal [iron] was subjected to more than 200 billion pascals of pressure".[105]
"[M]aterial within Earth's inner core is apparently distributed in a lopsided way ... The weakness of iron might lead crystallites in the inner core to flow and line up a certain way".[105]
"[T]he speed at which the inner core spun apparently fluctuated over the course of approximately decades between 1961 and 2007."[105]
"As the inner core cools, crystallizing iron releases impurities, sending lighter molten material into the liquid outer core. This upwelling, combined with the Earth's rotation, drives convection, forcing the molten metal into whirling vortices. These vortices stretch and twist magnetic field lines, creating Earth’s magnetic field. Currently, the center of the field, called an axis, emerges in the Arctic Ocean west of Ellesmere Island, about 300 miles (500 kilometers) from the geographic North Pole."[107]
"In the last decade, seismic waves from earthquakes revealed the inner core looks like a navel orange, bulging slightly more on its western half. Geoscientists recently explained the asymmetry by proposing a convective loop: The inner core might be crystallizing on one half and melting on the other."[107]
"The lopsided growth of the inner core makes convection in the outer core a little bit lopsided, and that then induces the geomagnetic field to have this lopsided or eccentric character too".[108]
"Magnetic particles trapped and aligned in rocks reveal that the magnetic north pole wandered around the Western Hemisphere over the past 10,000 years, and circled the Eastern Hemisphere before that — a result mirrored by the numerical test."[107]
"The key question for interesting ideas like translational instability is, 'Can we test it?' ... What we're doing is proposing a test, and we think it's a good test because people can go out and look for eccentricity in the rock record and that will either confirm or shoot down this idea."[108]
"Within less than 100 million years, everything that has been crystallized on the west will have melted on the east"[109]
Seismic "waves appear to travel faster through the inner core from north to south than from west to east. Seismic properties also seemed to vary between the Eastern and Western hemispheres of the globe."[110]
There is a "124-mile (200-km) thick layer of dense material detected on its surface."[110]
"[T]he inner core [may be] shifted slightly off-center, just to the east. This would put more pressure on the western side, where it would be closer to the center of the planet, and less pressure on the eastern side. The result could be a perpetually denser Western hemisphere and a continual flow of dense fluid from the east that eventually spreads out atop the entire inner core."[110]
"The inner core is basically regenerating itself. And superimposed on that is this overall cooling that makes the inner core bigger and bigger over time".[111]
"It is the first observational evidence that the inner core rotates at a variety of speeds with respect to the mantle...It also reconciles old discrepancies".[112]
"The inner core, on average, rotates eastward. At the speeds it travels, it might, on average, complete a revolution every 750 to 1,440 years. However, these speeds appear unstable, which makes it uncertain just how long it actually takes to finish a turn on its axis".[105]
Ceres
"Observations of 1 Ceres, the largest known asteroid, have revealed that the object may be a "mini planet," and may contain large amounts of pure water ice beneath its surface."[113]
"The observations by NASA's Hubble Space Telescope also show that Ceres shares characteristics of the rocky, terrestrial planets like Earth. Ceres' shape is almost round like Earth's, suggesting that the asteroid may have a "differentiated interior," with a rocky inner core and a thin, dusty outer crust."[113]
"Ceres is an embryonic planet."[113]
"Gravitational perturbations from Jupiter billions of years ago prevented Ceres from accreting more material to become a full-fledged planet."[113]
"Hubble snapped 267 images of Ceres. From those snapshots, the astronomers determined that the asteroid has a nearly round body. The diameter at its equator is wider than at its poles. Computer models show that a nearly round object like Ceres has a differentiated interior, with denser material at the core and lighter minerals near the surface. All terrestrial planets have differentiated interiors. Asteroids much smaller than Ceres have not been found to have such interiors."[113]
Europa
The darker regions are areas where Europa's primarily water ice surface has a higher mineral content. This surface is striated by cracks and streaks, while cratering is relatively infrequent. Other features present on Europa are circular and elliptical lenticulae (Latin for "freckles", reddish spots in the first image at left). Many are domes, some are pits and some are smooth, dark spots. Others have a jumbled or rough texture. The dome tops look like pieces of the older plains around them, suggesting that the domes formed when the plains were pushed up from below.[114] The prominent markings crisscrossing the moon seem to be mainly albedo features, which emphasize low topography.
"Reddish spots and shallow pits pepper the enigmatic ridged surface of Europa in this view combining information from images taken by NASA's Galileo spacecraft during two different orbits around Jupiter."[115]
"The spots and pits visible in this region of Europa's northern hemisphere are each about 10 kilometers (6 miles) across. The dark spots are called "lenticulae," the Latin term for freckles. Their similar sizes and spacing suggest that Europa's icy shell may be churning away like a lava lamp, with warmer ice moving upward from the bottom of the ice shell while colder ice near the surface sinks downward. Other evidence has shown that Europa likely has a deep melted ocean under its icy shell. Ruddy ice erupting onto the surface to form the lenticulae may hold clues to the composition of the ocean and to whether it could support life."[115]
"The image combines higher-resolution information obtained when Galileo flew near Europa on May 31, 1998, during the spacecraft's 15th orbit of Jupiter, with lower-resolution color information obtained on June 28, 1996, during Galileo's first orbit."[115]
Europa's most striking surface features are a series of dark streaks crisscrossing the entire globe, called lineae (lines). Close examination shows that the edges of Europa's crust on either side of the cracks have moved relative to each other. The larger bands are more than 20 km (12 mi) across, often with dark, diffuse outer edges, regular striations, and a central band of lighter material.[116]
The third image at the right is a "view of the Conamara Chaos region on Jupiter's moon Europa taken by NASA's Galileo spacecraft shows an area where the icy surface has been broken into many separate plates that have moved laterally and rotated. These plates are surrounded by a topographically lower matrix. This matrix material may have been emplaced as water, slush, or warm flowing ice, which rose up from below the surface. One of the plates is seen as a flat, lineated area in the upper portion of the image. Below this plate, a tall twin-peaked mountain of ice rises from the matrix to a height of more than 250 meters (800 feet). The matrix in this area appears to consist of a jumble of many different sized chunks of ice. Though the matrix may have consisted of a loose jumble of ice blocks while it was forming, the large fracture running vertically along the left side of the image shows that the matrix later became a hardened crust, and is frozen today. The Brooklyn Bridge in New York City would be just large enough to span this fracture."[117]
"North is to the top right of the picture, and the sun illuminates the surface from the east. This image, centered at approximately 8 degrees north latitude and 274 degrees west longitude, covers an area approximately 4 kilometers by 7 kilometers (2.5 miles by 4 miles). The resolution is 9 meters (30 feet) per picture element. This image was taken on December 16, 1997 at a range of 900 kilometers (540 miles) by Galileo's solid state imaging system."[117]
"This view [third down on the left] of Jupiter's icy moon Europa shows a region shaped like a mitten that has a texture similar to the matrix of chaotic terrain, which is seen in medium and high resolution images of numerous locations across Europa's surface. Development of such terrain may be one of the major processes for resurfacing the moon. North is to the top and the sun illuminates the surface from the left. The material in the "catcher's mitt" has the appearance of frozen slush and seems to bulge upward from the adjacent surface, which has been bent downward and cracked, especially along the southwest (lower left) margins. Scientists on the Galileo imaging team are exploring various hypotheses for the formation of such terrain including solid-state convection (vertical movement between areas which differ in density due to heating), upwelling of viscous icy "lava," or liquid water melting through from a subsurface ocean."[118]
"The image, centered at 20 degrees north latitude, 80 degrees west longitude covers an area approximately 175 by 180 kilometers (108 by 112 miles). The resolution is 235 meters per picture element. The images were taken on 31 May, 1998 Universal Time at a range of 23 thousand kilometers (14 thousand miles) by the Solid State Imaging (SSI) system on NASA's Galileo spacecraft."[118]
Protoplanetary disks
In December 2006, seven papers were published in the scientific journal, Science, discussing initial details of the sample analysis. Among the findings are: a wide range of organic compounds, including two that contain biologically usable nitrogen; indigenous aliphatic hydrocarbons with longer chain lengths than those observed in the diffuse interstellar medium; abundant amorphous silicates in addition to crystalline silicates such as olivine and pyroxene, proving consistency with the mixing of solar system and interstellar matter, previously deduced spectroscopically from ground observations;[119] hydrous silicates and carbonate minerals were found to be absent, suggesting a lack of aqueous processing of the cometary dust; limited pure carbon (CHON) was also found in the samples returned; methylamine and ethylamine was found in the aerogel but was not associated with specific particles.
Acknowledgements
The content on this page was first contributed by: Henry A. Hoff.
Initial content for this page in some instances came from Wikiversity.
See also
References
- ↑ Jane Beitler (2014). All About Snow. National Snow and Ice Data Center. Retrieved 2014-09-17.
- ↑ SemperBlotto (November 23, 2006). "geochemistry". San Francisco, California: Wikimedia Foundation, Inc. Retrieved 2013-08-29.
- ↑ cdm (2017). Tests for negative ions. UK: BBC. Retrieved 2017-04-11.
- ↑ SemperBlotto (10 September 2006). fluorescence. San Francisco, California: Wikimedia Foundation, Inc. Retrieved 2017-04-10.
- ↑ Principles Of Instrumental Analysis F.James Holler, Douglas A. Skoog & Stanley R. Crouch 2006
- ↑ Tom Harris. How Fluorescent Lamps Work. Discovery Communications. Retrieved 27 June 2010.
- ↑ Scott M. Juds (1988). Photoelectric sensors and controls: selection and application. CRC Press. p. 29. ISBN 978-0-8247-7886-6.
- ↑ Widsith (7 May 2011). specular. San Francisco, California: Wikimedia Foundation, Inc. Retrieved 2017-04-11.
- ↑ Dvortygirl (23 March 2006). luster. San Francisco, California: Wikimedia Foundation, Inc. Retrieved 2017-04-10.
- ↑ Kevin Ryde~enwiktionary (14 January 2006). glassy. San Francisco, California: Wikimedia Foundation, Inc. Retrieved 2017-04-10.
- ↑ Dvortygirl (14 June 2005). dull. San Francisco, California: Wikimedia Foundation, Inc. Retrieved 2017-04-11.
- ↑ Ncik (24 March 2005). metallic. San Francisco, California: Wikimedia Foundation, Inc. Retrieved 2017-04-11.
- ↑ brilliant. San Francisco, California: Wikimedia Foundation, Inc. 24 February 2017. Retrieved 2017-04-11.
- ↑ Paul G (29 November 2004). opaque. San Francisco, California: Wikimedia Foundation, Inc. Retrieved 2017-04-10.
- ↑ A. P. Meshik (2005). "The Workings of an Ancient Nuclear Reactor". Scientific American. Unknown parameter
|month=
ignored (help) - ↑ F. Gauthier-Lafaye; P. Holliger; P.-L. Blanc (1996). "Natural fission reactors in the Franceville Basin, Gabon: a review of the conditions and results of a "critical event" in a geologic system". Geochimica et Cosmochimica Acta. 60 (25): 4831–52. Bibcode:1996GeCoA..60.4831G. doi:10.1016/S0016-7037(96)00245-1.
- ↑ P. R. Fields, H. Diamond, D. N. Metta, D. J. Rokop, and C. M. Stevens (1972). "237Np, 236U, and other actinides on the moon". Proceedings of the Lunar Science Conference. 3: 1637–44. Bibcode:1972LPSC....3.1637F. Retrieved 2013-11-01.
- ↑ 18.0 18.1 K. Nassau (1976). "The deep blue Maxixe-type color center in beryl" (PDF). American Mineralogist. 61: 100.
- ↑ A. E. Champagne, A. J. Howard, and P. D. Parker (1983). "Nucleosynthesis of 26Al at low stellar temperatures". The Astrophysical Journal. 269 (06): 686–9. Bibcode:1983ApJ...269..686C. doi:10.1086/161077. Retrieved 2014-02-01. Unknown parameter
|month=
ignored (help) - ↑ Stokes, G. G. (1852). "On the Change of Refrangibility of Light". Philosophical Transactions of the Royal Society of London. 142: 463–562. doi:10.1098/rstl.1852.0022.
- ↑ K. Przibram (1935). "Fluorescence of Fluorite and the Bivalent Europium Ion". Nature. 135 (3403): 100. Bibcode:1935Natur.135..100P. doi:10.1038/135100a0.
- ↑ D.W. Thompson; et al. (1998). "Determination of optical anisotropy in calcite from ultraviolet to mid-infrared by generalized ellipsometry". Thin Solid Films. 313–4 (1–2): 341–6. Bibcode:1998TSF...313..341T. doi:10.1016/S0040-6090(97)00843-2.
- ↑ German Loan Words in English. German.about.com (2010-06-22). Retrieved on 2011-06-05.
- ↑ Essentials of Geology, 3rd Edition, Stephen Marshak, p 182
- ↑ Darryl Powell. Quartzite. Mineral Information Institute. Retrieved 2009-09-09.
- ↑ Deer, W.A.; Howie, R.A., & Zussman, J. (1992). An introduction to the rock forming minerals (2nd edition ed.). London: Longman ISBN 0-582-30094-0
- ↑ 27.0 27.1 Hurlbut, Cornelius S.; Klein, Cornelis (1985). Manual of Mineralogy, Wiley, (20th edition ed.). ISBN 0-471-80580-7
- ↑ Handbook of Mineralogy: Magnesioaxinite
- ↑ 29.0 29.1 http://rruff.geo.arizona.edu/doclib/hom/fluorapatite.pdf Mineral Handbook
- ↑ E. Skalwold. Pleochroism: trichroism and dichroism in gems. Nordskip.com. Retrieved 2011-08-29.
- ↑ YourGemologist / International School of Gemology Study of Heat Treatment. Yourgemologist.com. Retrieved 2011-08-29.
- ↑ H. Nechamkin, The Chemistry of the Elements, McGraw-Hill, New York, 1968.
- ↑ IMA Mineral List with Database of Mineral Properties
- ↑ 34.0 34.1 34.2 34.3 34.4 Essentials of Geology, 3rd Edition, Stephen Marshak
- ↑ http://rruff.geo.arizona.edu/doclib/hom/glaucophane.pdf Handbook of Mineralogy
- ↑ Farndon and Parker (2009). Minerals, Rocks and Fossils of the World. Lorenz Books
- ↑ http://rruff.info/ima
- ↑ 38.0 38.1 38.2 Gaines; et al. (1997). Dana’s New Mineralogy Eighth Edition. New York: Wiley.
- ↑ 39.0 39.1 Hauyne. Mindat.org. Retrieved 11 August 2011.
- ↑ Hauyne. Webminerals. Retrieved 11 August 2011.
- ↑ Handbook of Mineralogy
- ↑ Harvey, Eveline (14 January 2011). NZ blue ice sighting an unexpected treat for tourists, In: The New Zealand Herald. Retrieved 21 September 2011.
- ↑ Why Is Water Blue
- ↑ Color in the Beryl group. Retrieved 2009-06-06.
- ↑ Ibragimova, E. M., Mukhamedshina, N. M., A. Kh. Islamov (2009). "Correlations between admixtures and color centers created upon irradiation of natural beryl crystals". Inorganic Materials. 45 (2): 162. doi:10.1134/S0020168509020101.
- ↑ Viana, R. R., G. M. Da Costa, E. De Grave, W. B. Stern, H. Jordt-Evangelista (2002). "Characterization of beryl (aquamarine variety) by Mössbauer spectroscopy". Physics and Chemistry of Minerals. 29: 78. Bibcode:2002PCM....29...78V. doi:10.1007/s002690100210.
- ↑ Ana Regina Blak, Sadao Isotani and Shigueo Watanabe (1983). "Optical absorption and electron spin resonance in blue and green natural beryl: A reply". Physics and Chemistry of Minerals. 9 (6): 279. Bibcode:1983PCM.....9..279B. doi:10.1007/BF00309581.
- ↑ Malachite. WebExhibits. 2001. Retrieved 2007-12-08.
- ↑ (Oxford English Dictionary, 2nd Edition, Clarendon Press, Oxford, 1989.) See also first definition in Webster's New World Dictionary of the American Language, The World Publishing Company, New York, 1962.
- ↑ I. Bertini, N. Thomas, and C. Barbieri (2007). "Modeling of the light scattering properties of cometary dust using fractal aggregates". Astronomy & Astrophysics. 461 (1): 351–64. Bibcode:2007A&A...461..351B. doi:10.1051/0004-6361:20065461. Retrieved 2011-12-08. Unknown parameter
|month=
ignored (help); Unknown parameter|pdf=
ignored (help) - ↑ Susan L. Hallenbeck, Joseph A. Nuth III, and Robert N. Nelson (2000). "Evolving Optical Properties of Annealing Silicate Grains: from Amorphous Condensate to Crystalline Mineral". The Astrophysical Journal. 535 (1): 247–55. Retrieved 2013-11-19. Unknown parameter
|month=
ignored (help) - ↑ Webmineral
- ↑ 53.0 53.1 Handbook of Mineralogy
- ↑ MacEachern, Scott (1996) "Iron Age beginnings north of the Mandara Mountains, Cameroon and Nigeria" pp. 489–496 In Pwiti, Gilbert and Soper, Robert (editors) (1996) Aspects of African Archaeology: Proceedings of the Tenth Pan-African Congress University of Zimbabwe Press, Harare, Zimbabwe, ISBN 978-0-908307-55-5; archived here by Internet Archive on 11 March 2012
- ↑ Diop-Maes, Louise Marie (1996) "La question de l'Âge du fer en Afrique" ("The question of the Iron Age in Africa") Ankh 4/5: pp. 278–303, in French; archived here by Internet Archive on 25 January 2008
- ↑ Boswell, P. F. and Blanchard, Roland (1929) "Cellular structure in limonite" Economic Geology 24(8): pp. 791–796
- ↑ Northrop, Stuart A. (1959) "Limonite" Minerals of New Mexico (revised edition) University of New Mexico Press, Albuquerque, New Mexico, pp. 329–333 }}
- ↑ Constantinou, G. and Govett, G. J. S. (1972) "Genesis of sulphide deposits, ochre and umber of Cyprus" Transactions of the Institution of Mining and Metallurgy" 81: pp. 34–46
- ↑ M. E. Fleet and A. Hamid Mumin, Gold-bearing arsenian pyrite and marcasite and arsenopyrite from Carlin Trend gold deposits and laboratory synthesis, American Mineralogist 82 (1997) pp. 182–193
- ↑ Richard V. Gaines, H. Catherine W. Skinner, Eugene E. Foord, Brian Mason, and Abraham Rosenzweig: Dana's new mineralogy, p. 1106. John Wiley & Sons, 1997
- ↑ Smith, J.V. (1960) "The Crystal structure of Spurrite, Ca5(SiO4)2CO3". Acta. Cryst. 13, 454
- ↑ R. J. King (2002). "Minerals Explained 37: Cinnabar". Geology Today. 18 (5): 195–9. doi:10.1046/j.0266-6979.2003.00366.x.
- ↑ Ron Cowen (8 October 2009). Ice confirmed on an asteroid. Science News. Retrieved 9 October 2009.
- ↑ Nancy Atkinson (8 October 2009). More water out there, ice found on an asteroid, In: International Space Fellowship. Retrieved 11 October 2009.
- ↑ Campins, Humberto; Hargrove, K; Pinilla-Alonso, N; Howell, ES; Kelley, MS; Licandro, J; Mothé-Diniz, T; Fernández, Y; Ziffer, J (2010). "Water ice and organics on the surface of the asteroid 24 Themis". Nature. 464 (7293): 1320–1. doi:10.1039/nature09029. PMID 20428164.
- ↑ Rivkin, Andrew S.; Emery, Joshua P. (2010). "Detection of ice and organics on an asteroidal surface". Nature. 464 (7293): 1322–3. Bibcode:2010Natur.464.1322R. doi:10.1038/nature09028. PMID 20428165.
- ↑ More Water Out There, Ice Found on an Asteroid | International Space Fellowship
- ↑ R.W. Alexander Jr, R.J. Bell, M.A. Ordal, L.L. Long, M. Querry (1986). "Millimeter and submillimeter wavelength spectroscopy of natural minerals and metals". Applied Physics Communications. 6 (1). Retrieved 2013-10-20. Unknown parameter
|month=
ignored (help) - ↑ Samuel M Savin and Samuel Epstein (January 1970). "The oxygen and hydrogen isotope geochemistry of clay minerals". Geochimica et Cosmochimica Acta. 34 (1): 25–42. doi:10.1016/0016-7037(70)90149-3. Retrieved 28 May 2019.
- ↑ Ds. Lauretta, L.P. Keller, S. Messenger (2005). "Supernova olivine from cometary dust". Science. 309 (5735): 737–741. Bibcode:2005Sci...309..737M. doi:10.1126/science.1109602. PMID 15994379.
- ↑ Spitzer sees crystal 'rain' in outer clouds of infant star, Whitney Clavin and Trent Perrotto, Physorg.com, May 27, 2011 . Accessed May 2011
- ↑ 72.0 72.1 72.2 72.3 72.4 72.5 72.6 Kozo Sadakane and Minoru Ueta (1989). "Abundance Analysis of Sirius in the Blue-Violet Region". Publications of the Astronomical Society of Japan. 41 (2): 279–88. Bibcode:1989PASJ...41..279S. Unknown parameter
|month=
ignored (help);|access-date=
requires|url=
(help) - ↑ Cameron P. Wake, Paul Andrew Mayewski, Xie Zichu, Wang Ping, and Li Zhongquin (1993). "Regional Distribution of Monsoon and Desert Dust Signals Recorded in Asian Glaciers". Geophysical Research Letters. 20 (14): 1411–4. Retrieved 2014-09-29. Unknown parameter
|month=
ignored (help) - ↑ 74.0 74.1 74.2 T. Sivarani, P. Bonifacio, P. Molaro, R. Cayrel, M. Spite, F. Spite, B. Plez, J. Andersen, B. Barbuy, T. C. Beers, E. Depagne, V. Hill, P. François, B. Nordström, and F. Primas (2004). "First stars IV. CS 29497-030: Evidence for operation of the s-process at very low metallicity" (PDF). Astronomy and Astrophysics. 413 (1): 1073–85. arXiv:astro-ph/0310291. Bibcode:2004A&A...413.1073S. doi:10.1051/0004-6361:20031590. Retrieved 2012-06-02. Unknown parameter
|month=
ignored (help) - ↑ 75.0 75.1 75.2 75.3 75.4 75.5 Sarah K. Fortner, Bryan G. Mark, Jeffrey M. McKenzie, Jeffrey Bury, Annette Trierweiler, Michel Baraer, Patrick J. Burns, and LeeAnn Munk (2011). "Elevated stream trace and minor element concentrations in the foreland of receding tropical glaciers" (PDF). Applied Geochemistry. 26: 1792–1801. Retrieved 2014-09-30.
- ↑ 76.0 76.1 G. Stellmacher and E. Wiehr (1991). "Geometric line elevation in solar limb faculae". Astronomy and Astrophysics. 248 (1): 227–31. Bibcode:1991A&A...248..227S. Unknown parameter
|month=
ignored (help);|access-date=
requires|url=
(help) - ↑ H. L. Shipman (1977). "Masses, radii, and model atmospheres for cool white-dwarf stars". The Astrophysical Journal. 213 (4): 138–44. Bibcode:1977ApJ...213..138S. doi:10.1086/155138. Unknown parameter
|month=
ignored (help);|access-date=
requires|url=
(help) - ↑ P. Swings (1943). "Edlén's Identification of the Coronal Lines with Forbidden Lines of Fe X, XI, XIII, XIV, XV; Ni XII, XIII, XV, XVI; Ca XII, XIII, XV; a X, XIV". The Astrophysical Journal. 98 (07): 116–28. Bibcode:1943ApJ....98..116S. doi:10.1086/144550. Unknown parameter
|month=
ignored (help);|access-date=
requires|url=
(help) - ↑ 79.0 79.1 79.2 D. Van Rooij, N. Zaazi, N. Fagel, M. Boone, V. Cnudde, J. Dewanckele, H. Pirlet, U. Rohl, D. Blamart, J.-P. Henriet, P. Jacobs, H. Houbrechts, P. Duyck, and R. Swennen (2009). "3D anatomy of Heinrich Layer 2" (PDF). Geophysical Research Abstracts. 11 (EGU2009-4809–1): 1. Retrieved 2014-09-29.
- ↑ G. Catanzaro (2010). "First spectroscopic analysis of β Scorpii C and β Scorpii E Discovery of a new HgMn star in the multiple system β Scorpii" (PDF). Astronomy & Astrophysics. 509: 7. doi:10.1051/0004-6361/200913332. Retrieved 2013-01-18. Unknown parameter
|month=
ignored (help) - ↑ Kent S. Whiting and Gunter Faure (1991). "Transport of Magnetite and Ilmenite by Glaciers in the Adirondack Mountains of New York". The Journal of Geology. 99 (3): 482–92. Retrieved 2014-10-01. Unknown parameter
|month=
ignored (help) - ↑ Drever, James (2002). The Geochemistry of Natural Waters. New Jersey: Prentice Hall. pp. 311–322. ISBN 0-13-272790-0.
- ↑ 83.0 83.1 Avner Vengosh, Allan R Chivas, Malcolm T McCulloch, Abraham Starinsky, Yehoshua Kolodny (1991). "Boron isotope geochemistry of Australian salt lakes". Geochimica et Cosmochimica Acta. 55 (9): 2591–606. Retrieved 2013-08-29. Unknown parameter
|month=
ignored (help) - ↑ Georges Audi, O. Bersillon,J. Blachot, and A.H. Wapstra (2003). "The NUBASE Evaluation of Nuclear and Decay Properties". Nuclear Physics A. Atomic Mass Data Center. 729 (1): 3–128. Bibcode:2003NuPhA.729....3A. doi:10.1016/j.nuclphysa.2003.11.001.
- ↑ W. W. Strong (1909). "On the Possible Radioactivity of Erbium, Potassium and Rubidium". Physical Review (Series I). 29 (2): 170–3. Bibcode:1909PhRvI..29..170S. doi:10.1103/PhysRevSeriesI.29.170.
- ↑ David R Lide, H. P. R. Frederikse (June 1995). CRC handbook of chemistry and physics: a ready-reference book of chemical and physical data. pp. 4–25. ISBN 978-0-8493-0476-7.
- ↑ Doane, TA (2017). "A survey of photogeochemistry". Geochem Trans. 18: 1. doi:10.1186/s12932-017-0039-y. PMC 5307419. PMID 28246525.
- ↑ Ferris, F.G. (2005). "Biogeochemical properties of bacteriogenic iron oxides". Geomicrobiology Journal. 22: 79–85. doi:10.1080/01490450590945861.
- ↑ Spiro, T.G.; Bargar, J.R.; Sposito, G; Tebo, B.M. (2010). "Bacteriogenic manganese oxides". Accounts of Chemical Research. 43: 2–9. doi:10.1021/ar800232a.
- ↑ Aquatic Ecosystems: Interactivity of Dissolved Organic Matter. Academic Press. 2002.
- ↑ Moore, Benjamin (1912). The Origin and Nature of Life. Williams and Norgate. p. 182.
- ↑ Xu, Y; Schoonen, MAA (2000). "The absolute energy positions of conduction and valence bands of selected semiconducting minerals". American Mineralogist. 85: 543–556. Bibcode:2000AmMin..85..543X. doi:10.2138/am-2000-0416.
- ↑ Kisch, Horst (2015). Semiconductor photocatalysis: principles and applications. Wiley. ISBN 978-3-527-33553-4.
- ↑ Sue Lavoie (6 October 2008). PIA11389: A Patch of Black. Pasadena, California USA: NASA/JPL. Retrieved 2015-02-03.
- ↑ Christina Dorr and Julie Taylor (29 September 2009). Seeing Double?. Baltimore, Maryland USA: Johns Hopkins University Applied Physics Laboratory. Retrieved 2015-02-03.
- ↑ Colleen Schroeder (10 May 2005). Hills of Mercury. Pasadena, California USA: NASA/JPL. Retrieved 2015-02-03.
- ↑ 97.0 97.1 Northwestern University (18 January 2000). PIA02446: Discovery Scarp. Northwestern University. Retrieved 2016-11-07.
- ↑ Theodore E. Madey, Robert E. Johnson, Thom M. Orlando (2002). "Far-out surface science: radiation-induced surface processes in the solar system" (PDF). Surface Science. 500 (1–3): 838–58. doi:10.1016/S0039-6028(01)01556-4. Retrieved 2012-02-09. Unknown parameter
|month=
ignored (help) - ↑ Charles Schlemm, Richard D. Starr, George C. Ho, Kathryn E. Bechtold, Sarah A. Hamilton, John D. Boldt, William V. Boynton, Walter Bradley, Martin E. Fraeman and Robert E. Gold; et al. (2007). "The X-Ray Spectrometer on the MESSENGER Spacecraft". Space Science Reviews. 131 (1): 393–415. Bibcode:2007SSRv..131..393S. doi:10.1007/s11214-007-9248-5.
|access-date=
requires|url=
(help) - ↑ X-ray Spectrometer (XRS). NASA / National Space Science Data Center. Retrieved 2011-02-19.
- ↑ Jean-Paul Poirier (2000). Introduction to the Physics of the Earth's Interior. Cambridge Topics in Mineral Physics & Chemistry. Cambridge University Press. ISBN 0-521-66313-X.
- ↑ Aimin Cao (April 14, 2005). Finally, a Solid Look at Earth's Core. Live Science. Retrieved 2013-05-14.
- ↑ Robert Roy Britt (April 14, 2005). Finally, a Solid Look at Earth's Core. Live Science. Retrieved 2013-05-14.
- ↑ William Lowrie (2004). Fundamentals of Geophysics. Cambridge University Press. ISBN 0-521-46164-2.
- ↑ 105.0 105.1 105.2 105.3 105.4 Charles Q. Choi (May 13, 2013). Earth's Rotating Inner Core Shifts Its Speed. Yahoo! News. Retrieved 2013-05-14.
- ↑ Arianna Gleason (May 13, 2013). Earth's Rotating Inner Core Shifts Its Speed. Yahoo! News. Retrieved 2013-05-14.
- ↑ 107.0 107.1 107.2 Becky Oskin (July 18, 2012). Why Earth's Magnetic Field Is Wonky. LiveScience. Retrieved 2013-05-14.
- ↑ 108.0 108.1 Peter Olson (July 18, 2012). Why Earth's Magnetic Field Is Wonky. LiveScience. Retrieved 2013-05-14.
- ↑ Thierry Alboussiere (August 4, 2010). Earth's Inner Core Might Be on the Move. Live Science. Retrieved 2013-05-14.
- ↑ 110.0 110.1 110.2 Lynne Peeples (August 4, 2010). Earth's Inner Core Might Be on the Move. Live Science. Retrieved 2013-05-14.
- ↑ Michael Bergman (August 4, 2010). Earth's Inner Core Might Be on the Move. Live Science. Retrieved 2013-05-14.
- ↑ Hrvoje Tkalcic (May 13, 2013). Earth's Rotating Inner Core Shifts Its Speed. Yahoo! News. Retrieved 2013-05-14.
- ↑ 113.0 113.1 113.2 113.3 113.4 Lucy A. McFadden (7 September 2005). Largest Asteroid May Be 'Mini Planet' with Water Ice. Baltimore, Maryland USA: HubbleSite. Retrieved 2015-02-04.
- ↑ Sotin, Christophe; Head III, James W.; and Tobie, Gabriel (2001). Europa: Tidal heating of upwelling thermal plumes and the origin of lenticulae and chaos melting (PDF). Retrieved 2007-12-20.
- ↑ 115.0 115.1 115.2 Sue Lavoie (October 30, 2002). PIA03878: Ruddy "Freckles" on Europa. Washington, D.C. USA: NASA's Office of Space Science. Retrieved 2013-06-24.
- ↑ Geissler, Paul E.; Greenberg, Richard; et al. (1998). Evolution of Lineaments on Europa: Clues from Galileo Multispectral Imaging Observations. Retrieved 2007-12-20.
- ↑ 117.0 117.1 Sue Lavoie (March 2, 1998). PIA01177: Chaotic Terrain on Europa in Very High Resolution. Washington, DC USA: NASA's Office of Space Science. Retrieved 2013-06-24.
- ↑ 118.0 118.1 Sue Lavoie (October 13, 1998). PIA01640: Mitten shaped region of Chaotic Terrain on Europa. Washington, DC USA: NASA's Office of Space Science. Retrieved 2013-06-24.
- ↑ The building blocks of planets within the `terrestrial' region of protoplanetary disks. nottingham.ac.uk. Retrieved 2008-03-04.
External links
{{Principles of radiation astronomy}}
Editor-In-Chief: Henry A Hoff
- Pages with citations using unsupported parameters
- CS1 maint: Multiple names: authors list
- CS1 maint: Explicit use of et al.
- Pages using citations with accessdate and no URL
- Pages with broken file links
- Astrochemistry lectures
- Minerals/Lectures
- Radiation astronomy lectures
- Resources last modified in October 2019